Chapter 1: Introduction – Advanced Fabricated Building
Building codes are sets of regulations governing the design, construction, alteration, and maintenance of structures. They specify the minimum requirements for adequately safeguarding the health, safety, and welfare of building occupants. For the purposes of this course we will focus on two building codes: (1) the Manufactured Home Construction and Safety Standards or HUD Code (see Figure 1.1) which applies to manufactured homes under a program administered by the US Department of Housing and Urban Development (HUD) under a program administered by the US Department of Housing and Urban Development (HUD), and the (2) the International Residential Code or IRC (see Figure 1.1) which applies to modular buildings (see Figure 1.1). In addition to these two codes that govern the construction of manufactured and modular homes, the Energy Code that spells out the requirements for energy conserving features for the modular home will be covered. The HUD Code includes the energy standards for manufactured homes. In Florida, the Florida Building Code (2017) Energy Conservation specifies the energy standards and requirements for modular homes.
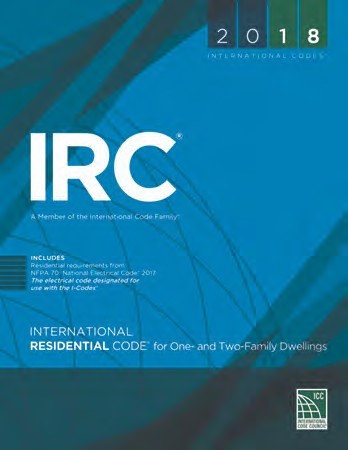
1.1.1 Today’s Major Building Codes
Rather than create and maintain their own codes, most states and local jurisdictions adopt the model building codes maintained by the International Code Council (ICC). In 2000, the three regionally-based model code organizations (BOCA Standard National Code, SBCCI Standard Code, and ICBO Uniform Code) combined together to form the ICC. The ICC family of International Codes includes:
- International Building Code (IBC): applies to all types of new buildings
- International Residential Code (IRC): applies to new one-and two-family dwellings and townhouses of not more than three stories in height
- International Existing Building Code (IEBC): applies to the alteration, repair, or change in occupancy of existing structures.
The ICC publishes new additions of the International Codes every three years and many states and local jurisdictions have adopted them since the first editions were issued in 2000.
Modular homes manufactured in factories are subject to the same regulations and standards as site built homes. The basic difference between inspections of site built homes and manufactured modular homes is that for modular homes, the inspections are conducted by state regulators during the manufacturing process and again after delivery to the site and the installation of the home on its foundation.
Manufactured homes or HUD homes are subject to federal inspections during the in plant manufacturing process and to inspections by the local jurisdiction during the on-site installation process (see Figure 1.2). Manufacturers are held accountable for building homes that are compliant with HUD’s standards by agents of HUD who are referred to as Production Inspection and Primary Inspection Agencies (IPIA). The IPIA is responsible for certifying manufacturing plants before they can begin operations and for routinely inspecting manufacturing in the plants to ensure that they are in compliance with the HUD Code. There are 10 states, including Florida, in which a state agency acts as the IPIA. In the other states where mobile/manufactured home manufacturing occurs, the IPIA is a private company. In Florida, the IPIA is the Bureau of Manufactured Homes/Recreational Vehicle Construction, located in the Division of Motor Vehicles in Tallahassee, Florida.
Figure 1.2 Every mobile/manufactured home must receive a HUD label certifying that it was built in accordance with the Federal Manufactured Housing Construction and Safety Standards (HUD Code). (Source: US Department of Housing and Urban Development)
1.1.2 – Early History of Building Codes
The earliest known written building code was part of the Code of Hammurabi which dates from about 1754 BC. This Code embodied the laws of ancient Mesopotamia and included 282 laws that specify punishments for a wide variety of infractions including harm caused by a poorly constructed home or building. Among the building related provisions in the Code Of Hammurabi are the following (see Figure 1.3):
- If a builder builds a house for someone and completes it, he shall give him a fee of two shekels in money for each “sar” of service. A shekel was the basic monetary unit and it had a weight of 8.33 grams of silver, worth about $32.50 today. Note: A sar is a unit of area that was about 400 square feet in size.
- If a builder builds a house for someone and does not construct it properly, and the house which he builds falls in and kills its owner, then that builder shall be put to death.
- If it kills the son of the owner, the son of that builder shall be put to death.
- If it kills a slave of the owner, then he shall pay slave for slave to the owner of the house.
- If it ruins goods, he shall make compensation for all that has been ruined, and inasmuch as he did not construct the house properly and it collapses, he shall re-erect the house from his own means.
- If a builder builds a house for someone, even though he has not yet completed, if the walls appear to be about to topple, the builder must make the walls solid from his own means.
Figure 1.3 The Code of Hammurabi was the law code of the ancient city of Babylon and copies of the code were etched into man-sized clay tablets for the citizens to read. (Source: Wikipedia Commons)
Mention of code requirements for buildings can be found in the book of Deuteronomy in the Bible in which it is stipulated that parapets must be constructed on all houses to prevent people from falling off. Another mention of building code requirements in the Bible can be found in Leviticus where the methodology on how to treat toxic mold and a system of inspection were established:
On the seventh day the priest shall return to inspect the house. If the mildew has spread on the walls, he is to order that the contaminated stones be torn out and thrown into an unclean place outside the town. He must have all the inside walls of the house scraped and the material that is scraped off dumped into the unclean place outside of town. Then they are to take other stones to replace these and take new clay and plaster the house. If the mildew reappears in the house after the stones have been torn out and the house is scraped and plastered, the priest is to go and examine it and, if the mildew has spread in the house, it is a destructive mildew, the house is unclean. It must be torn down, its stones timbers and all the plaster must be taken out of the town to the unclean place.
In the Middle Ages, the Great Fire of London in 1666 resulted in the first modern era building code, known as the Rebuilding of London Act (see Figure 1.4). The fire destroyed over 13,000 homes plus St Paul’s Cathedral and the result was that over 70,000 residents of London were left homeless. The enormous damage caused by this fire was a result of a combination of a dense built environment, oak timber and tar construction, narrow streets, and primitive fire fighting methods such as the bucket brigades which passed buckets of water from water sources to the fire. The Act required that housing must have some fire resistance and it also regulated the construction of the City of London by, for example, regulating the width of roads.
Figure 1.4 The Great Fire of London in 1666 was the triggering event for the enactment of the first known building codes. (Source: UK Government)
The London Building Act of 1844 was the first real national building standard. It contains provisions such as the thickness of walls, the height of rooms, the materials used in construction, and the placing and design of chimneys, fireplaces, and drains. The Metropolitan Buildings Office was formed to regulate construction throughout London and surveyors were empowered to enforce building regulations.
1.1.3 History of U.S. Building Codes
In early America, George Washington and Thomas Jefferson encouraged the development of building regulations to provide minimum standards that would ensure health and safety. The key events in the evolution of building codes in the United States include the following:
1631 – City of Boston fire prevention ordinance bans wood chimneys and thatch roof coverings.
1678 – City of Boston building laws require slate or tile roofs and brick walls.
1788 – Old Salem (now Winston-Salem, NC) writes first known formal US Building Code.
1844 – The London Building Act of 1844 required drainage improvements, sufficient street widths to ensure adequate ventilation, regulation of explosives, and required inspectors be appointed to supervise the act. The Metropolitan Building Office was established in 1845.
1859 – Baltimore passes its first building code.
1865 – New Orleans enacts a law requiring inspections of public places.
1867 – Due to the large influx of immigrants over the years and barely tolerable living conditions in tenement buildings, the City of New York enacts a “Tenement Housing Act”. This act requires fire escapes and a window for every room.1875 – As a result of the Chicago fire of 1871 the City of Chicago enacts ordinances regulating building construction and fire prevention.
One of the first building modern era building codes, the National Building Code, was developed in 1905 by the Fire Underwriters Association and with the intent that it become a national code. It was directed towards protecting the building rather than the people in the building. National codes that focused on the safety of the occupants (fire exiting, fire alarms, isolation of hazards) were not developed until the 1930’s and 1940’s. The creation of the National Building Code led to the formation of organizations of building officials. By 1940, the United States had three regional code organizations, each with its own code: the BOCA Standard National Code, the SBCCI Standard Code, and the ICBO Uniform Code. As noted earlier, these three organizations and their codes were consolidated into the International Code Council (ICC) and the first set of “I-codes” was published in 2000. These codes include the International Building Code (IBC), the International Residential Code (IRC), the International Energy Conservation Code (IECC), as well as mechanical, plumbing, fire and other codes.
Chapter 1.2: The Manufactured Home Construction and Safety Standard (HUD Code)- Advanced Fabricated Building
In the U.S. a manufactured home (formerly known as a mobile home) must be built to the Manufactured Home Construction and Safety Standards (HUD Code) which was developed in 1976. The U.S. Department of Housing and Urban Development (HUD) began to regulate the construction of mobile/manufactured homes in 1976 pursuant to the provisions of the National Manufactured Housing Construction and Safety Standards Act of 1974. As a consequence of the extensive damage done to manufactured homes by Hurricane Andrew in 1991, HUD amended the Manufactured Housing Construction and Safety Standards in 1994 to provide for greater protection for mobile/manufactured homes from wind damage. During the severe hurricane seasons of 2004 and 2005, no mobile/manufactured home that was built after these new standards went into effect sustained any significant structural damage from the storms.
A manufactured home displays a red certification label on the exterior of each transportable section. Manufactured homes are built in the controlled environment of a manufacturing plant and are transported in one or more sections on a permanent chassis. Manufactured homes are constructed according to a code administered by the U.S. Department of Housing and Urban Development (HUD Code). The HUD Code, unlike conventional building codes, requires manufactured homes to be constructed on a permanent chassis.
1.2.1 HUD Code Definitions
Certification label means the approved form of certification by the manufacturer that is permanently affixed to each transportable section of each manufactured home manufactured for sale in the United States.
Figure 1.5 The Certification Label must be permanently attached to every manufactured home sold in the U.S.
Certification report: the report prepared by an IPIA (see definition below) for each manufactured home manufacturing plant in which the IPIA provides a complete description of the initial comprehensive inspection of the plant, an evaluation of the quality assurance program under the approved quality assurance manual, and the identity of the DAPIA (see definition below) which approved the designs and quality assurance manual used in the plant. Where appropriate the certification report may be made by a DAPIA.
Date of manufacture: the date on which the required label is affixed to the manufactured home.
Defect: a failure to comply with an applicable Federal manufactured home safety and construction standard that renders the manufactured home or any part or component thereof not fit for the ordinary use for which it was intended, but does not result in an unreasonable risk of injury or death to occupants of the affected manufactured home.
Design: drawings, specifications, sketches and the related engineering calculations, tests and data in support of the configurations, structures and systems to be incorporated in manufactured homes manufactured in a plant.
Design Approval PIA (DAPIA): an agency that evaluates and approves or disapproves manufactured home designs and quality control procedures.
Failure to conform: an imminent safety hazard related to the standards, a serious defect, defect, or noncompliance and is used as a substitute for all of those terms.
Federal Manufactured Home Construction and Safety Standard: a reasonable standard for the construction, design, and performance of a manufactured home which meets the needs of the public including the need for quality, durability, and safety. Also referred to as the HUD Code HUD Display Label: Every mobile/manufactured home must receive a HUD label certifying that it was built in accordance with the Federal Manufactured Housing Construction and Safety Standards. The label shall be approximately 2 inches by 4 inches in size and shall be permanently attached to the home by means of 4 blind rivets, drive screws, or other means that render it difficult to remove without defacing it. It shall be etched on 0.32 inches thick aluminum plate. The label number shall be etched or stamped with a 3 letter designation which identifies the Production Inspection and Primary Inspection Agency (IPIA) for the state in which the home is manufactured. Each label shall also be marked with a 6 digit number which the label supplier will furnish. The label shall be located at the tail–light end of each transportable section of the home approximately one foot up from the floor and one foot in from the road side.
Imminent safety hazard: a hazard that presents an imminent and unreasonable risk of death or severe personal injury that may or may not be related to failure to comply with an applicable Federal manufactured home construction or safety standard.
Installations: all arrangements and methods of construction, as well as fire safety, plumbing, heat-producing and electrical systems used in manufactured homes. recognized standards or tests to determine suitable usage in a specified manner.
Label or certification label: the approved form of certification by the manufacturer that is permanently affixed to each transportable section of each manufactured home manufactured for sale to a purchaser in the United States.
Length of a manufactured home: the largest overall length in the traveling mode for a manufactured home, including cabinets and other projections which contain interior space. Length does not include bay windows, roof projections, overhangs, or eaves under which there is no interior space, nor does it include drawbars, couplings or hitches.
Manufactured home: a structure, transportable in one or more sections, which in the traveling mode is 8 body feet or more in width or 40 body feet or more in length or which when erected on-site is 320 or more square feet, and which is built on a permanent chassis and designed to be used as a dwelling with or without a permanent foundation when connected to the required utilities, and includes the plumbing, heating, air-conditioning, and electrical systems contained in the structure. The term does not include any self-propelled recreational vehicle. Calculations used to determine the number of square feet in a structure will include the total of square feet for each transportable section comprising the completed structure and will be based on the structure’s exterior dimensions measured at the largest horizontal projections when erected on site. These dimensions will include all expandable rooms, cabinets, and other projections containing interior space, but do not include bay windows.
Noncompliance: a failure of a manufactured home to comply with a Federal manufactured home construction or safety standard that does not constitute a defect, serious defect, or imminent safety hazard.
Production Inspection PIA (IPIA): an agency that evaluates the ability of manufactured home manufacturing plants to follow approved quality control procedures and provides ongoing surveillance of the manufacturing process.
Quality Assurance Manual: a manual, prepared by each manufacturer for its manufacturing plants and approved by a DAPIA which contains: a statement of the manufacturer’s quality assurance program, a chart of the organization showing, by position, all personnel accountable for quality assurance, a list of tests and test equipment required, a station-by-station description of the manufacturing process, a list of inspections required at each station, and a list by title of personnel in the manufacturer’s organization to be held responsible for each inspection. Where necessary, the quality assurance manual used in a particular plant shall contain information specific to that plant.
Red tag: affixing a notice to a manufactured home which has been found to contain an imminent safety hazard or a failure to conform with any applicable standard. A red tag is the notice so affixed to the manufactured home.
Serious defect: any failure to comply with an applicable Federal manufactured home construction and safety standard that renders the manufactured home or any part thereof not fit for the ordinary use for which it was intended and which results in an unreasonable risk of injury or death to occupants of the affected manufactured home.
Width of a manufactured home: the largest overall width of a manufactured home in the traveling mode, including cabinets and other projections which contain interior space. Width does not include bay windows, roof projections, overhangs, or eaves under which there is no interior space.
1.2.2 Major Provisions of the HUD Code
HUD Wind Zones and Thermal Zones
A HUD home is designed and built to be wind resistant and energy efficient. Wind resistance is provided by metal hurricane straps that connect the roof, walls, and floor together and to the foundation or ground. Homes that will be located in zones with higher winds will have stronger and more robust systems to tie the home to its foundation. For instance, a home located in coastal Louisiana must be more wind-resistant than one in Kentucky.
Similarly, the colder the climate the more insulation and thermal resistance is required for the home envelope. Homes located in Michigan will require higher envelope thermal resistance than homes located in Florida.
The HUD Code specifies wind and thermal resistance requirements based on dividing the U.S. into zones. There are three wind zones and three thermal resistance zones and the division of the country is different for the wind zones compared to the thermal zones. The wind and thermal requirements and their corresponding zones are described in the following sections.
HUD Wind Zones
Each manufactured home must be designed according to the federal HUD Code. The HUD Code stipulate that the home shall be designed and constructed to conform to one of three wind load zones. The higher the number of the Wind Zone, the more stringent are the requirements for wind-resistant construction. The appropriate Wind Zone used in design is dependent on where the home will be initially installed. Homes designed and constructed to a higher Wind Zone can be installed in a lower Wind Zone (a Wind Zone III home can be installed in a Wind Zone I or II location). However, a Wind Zone I home cannot be installed in either a Wind Zone II or III area.
Wind loads are lateral loads that must be resisted by the home. The home must be capable of transferring these imposed lateral loads to the home’s stabilizing devices without exceeding the allowable stresses and other deflection requirements. Wind Zone I, Wind Zone II and Wind Zone III are identified on the basic Wind Zone Map below. The manufactured home producer designs the home to resist the wind load, which is measured in pounds per square foot. Wind Zone I equates to a 70-mph fastest-mile wind speed; Wind Zone II to a 100-mph fastest-mile wind speed; and Wind Zone III to a 110-mph fastest-mile wind speed. Most of the US is in Wind Zone I except for Gulf and Atlantic coastal areas. Wind Zone III is a small area in south Florida that includes Miami and the Florida Keys plus portions of Louisiana.
Figure 1.6 There are three Wind Zones in the HUD Code, with Wind Zone III designating the highest wind velocities. The higher the Wind Zone, the more stringent are the requirements for connecting the home and it components together and to the ground.
HUD Thermal Zones
In addition to the Wind Zones, HUD specifies three Thermal Zones that designate varying levels of thermal resistance for the home envelope. The higher the Thermal Zone number, the more thermal resistance or insulation is required. The Thermal Zones are shown below together with the minimum required Uo value. Uo indicates how much resistance the wall, window, or roof must have to energy flows between the outside and home interior. The lower the Uo value the more thermal resistance the envelope must have. The further north the home will be installed, the lower the Uo value must be to provide adequate thermal resistance for the purpose or reducing energy consumption and cost. In simple terms, a lower Uo value indicates the home envelope has more insulation. Note that a higher number Wind Zone has a lower Uo value.
Figure 1.7 The HUD Code requires more insulation and thermal resistance for homes in the colder northern U.S. compared to the south. Thermal Zone 3 is the most stringent area and requires the most insulation due to the large temperature differences that occur between inside and outside the home. The Uo value is a measure of thermal resistance and a lower number indicates more resistance.
The appropriate Thermal Zone value used in design is dependent on where the home will be initially installed. Homes designed and constructed to a higher Thermal Zone can be installed in a lower Thermal Zone (a home designed to a Thermal Zone 3 can be installed in a Thermal Zone 1 or 2). However, a Thermal Zone 1 home cannot be installed in either a Thermal Zone 2 or 3 area.
The Uo value is the overall coefficient of heat transmission of the manufactured home based on the respective thermal zone location and an indoor design temperature of 70 oF, and is defined in units of BTU/(hour)(square foot)(oF). The overall Uo value for the home thermal envelope design must not exceed the values as shown on the above map. The design Uo value for Thermal Zone 1 is 0.116 BTU/hr·ft2·oF, for Thermal Zone 2 is 0.096 BTU/ hr·ft2·oF, and for Thermal Zone 3 is 0.079 BTU/ hr·ft2·oF. Again, the lower the Uo value, the greater the thickness of insulation that is required.
1.2.2.2 Major HUD Code Requirements
1.2.2.2.1 Lighting and Ventilation
Lighting. Each habitable room shall be provided with exterior windows and/or doors having a total glazed area of not less than 8 percent of the gross floor area.
- Kitchens, bathrooms, toilet compartments, laundry areas, and utility rooms may be provided with artificial light in place of
- Rooms and areas may be combined for the purpose of providing the required natural lighting provided that at least one half of the common wall area is open and unobstructed, and the open area is at least equal to 10 percent of the combined floor area or 25 square feet whichever is greater.
Whole-house ventilation. Each manufactured home must be provided with whole-house ventilation having a minimum capacity of 0.035 cubic feet per minute (cfm)/ft2 of interior floor space or its hourly average equivalent. This ventilation capacity must be in addition to any openable window area. In no case shall the installed ventilation capacity of the system be less than 50 cfm nor more than 90 cfm.
- The ventilation capacity must be provided by a mechanical system or a combination passive and mechanical system. Mechanical means fan powered and passive refers to ventilation cby air flow that is caused by natural forces such as the chimney effect in which air rises due to warming. Passive implies a fan is not being used. A combination passive and mechanical system can operate in either
- The ventilation system or provisions must exchange air directly with the exterior of the home, except that the ventilation system must not draw or expel air from or to the space underneath the home. The ventilation system must not draw or expel air into the floor, wall, or ceiling/roof systems, even if those systems are vented. The ventilation system must be designed to ensure that outside air is distributed to all bedrooms and main living areas. The combined use of undercut doors or transom grills connecting those areas to the room where the mechanical system is located meets this requirement.
- The ventilation system or a portion of the system is permitted to be integral with the home’s heating or cooling system. The system must be capable of operating independently of the heating or cooling modes. A ventilation system that is integral with the heating or cooling system is to be listed as part of the heating and cooling system or listed as suitable for use with that
- A mechanical ventilation system, or mechanical portion thereof, must be provided with a manual control, and must be permitted to be provided with automatic timers or
- A whole-house ventilation label must be attached to the whole-house ventilation control, must be permanent, and must state: ‘‘WHOLE-HOUSE ’’
Additional ventilation.
- At least half of the minimum required glazed area in paragraph (a) above shall be openable directly to the outside of the manufactured home for unobstructed
- Kitchens shall be provided with a mechanical ventilation system that is capable of exhausting 100 cfm to the outside of the home. The exhaust fan shall be located as close as possible to the range or cook top, but in no case farther than 10 feet horizontally from the range or cook
- Each bathroom and separate toilet compartment shall be provided with a mechanical ventilation system capable of exhausting 50 cfm to the outside of the home. A separate toilet compartment may be provided with 1.5 square feet of openable glazed area in place of mechanical ventilation, except in Uo value Zone
1.2.2.2.2 Exit Facilities and Exterior Doors.
Number and location of exterior doors. Manufactured homes shall have a minimum of two exterior doors located remote from each other.
- Required egress doors shall not be located in rooms where a lockable interior door must be used in order to
- In order for exit doors to be considered remote from each other, they must comply with all of the following:
- Both of the required doors must not be in the same room or in group of rooms which are not defined by fixed
- Single wide units: Doors may not be less than 12 ft. from each other as measured in any straight line direction regardless of the length of path of travel between
- Double wide units: Doors may not be less than 20 ft. from each other as measured in any straight line direction regardless of the length of path of travel between
- One of the required exit doors must be accessible from the doorway of each bedroom without traveling more than 35
1.2.2.2.3 Egress Windows and Devices.
- Every room designed expressly for sleeping purposes, unless it has an exit door, shall have at least one outside window or approved exit
- The bottom of the window opening shall not be more than 36 inches above the
- Locks, latches, operating handles, tabs, and any other window screen or storm window devices which need to be operated in order to permit exiting, shall not be located in excess of 54 inches from the finished
- Integral rolled-in screens shall not be permitted in an egress window unless the window is of the hinged
1.2.2.2.4 Room Requirements.
- Every manufactured home shall have at least one living area with not less than 150 sq. ft. of gross floor area.
- Rooms designed for sleeping purposes shall have a minimum gross square foot floor area as follows:
- All bedrooms shall have at least 50 sq. ft. of floor
- Bedrooms designed for two or more people shall have 70 sq. ft. of floor area plus 50 sq. ft. for each person in excess of
- Every room designed for sleeping purposes shall have accessible clothes hanging space with a minimum inside depth of 22 inches and shall be equipped with a rod and
1.2.2.2.5 Toilet Compartments.
Each toilet compartment shall be a minimum of 30 inches in width, except, when the toilet is located adjacent to the short dimension of the tub, the distance from the tub to the center line of the toilet shall not be less than 12 inches. At least 21 inches of clear space shall be provided in front of each toilet.
1.2.2.2.6 Hallways.
Hallways shall have a minimum horizontal dimension of 28 inches measured from the interior finished surface to the interior finished surface of the opposite wall. When appliances are installed in a laundry area, the measurement shall be from the front of the appliance to the opposite finished interior surface. When appliances are not installed and a laundry area is provided, the area shall have a minimum clear depth of 27 inches in addition to the 28 inches required for passage. In addition, a notice of the available clearance for washer/dryer units shall be posted in the laundry area. Minor protrusions into the minimum hallway width by doorknobs, trim, smoke alarms or light fixtures are permitted.
1.2.2.2.7 Glass and glazed openings.
Windows and sliding glass doors. All windows and sliding glass doors shall meet the requirements of the ‘‘Standard for Windows and Sliding Glass Doors Used in Manufactured Homes’’.
Safety glazing. Glazing in all entrance or exit doors, sliding glass doors, units (fixed or moving sections), unframed glass doors, unbacked mirrored wardrobe doors (i.e., mirrors not secured to a backing capable of being the door itself), shower and bathtub enclosures and surrounds to a height of 6 feet above the bathroom floor level, storm doors or combination doors, and in panels located within 12 inches on either side of exit or entrance doors shall be of a safety glazing material. Safety glazing material is considered to be any glazing material capable of passing the requirements of the governing standards.
1.2.2.2.8 Fire Safety and Flame Spread Requirements
Materials are rated with respect to their fire safety by the Flame Spread Index. The Flame Spread Rating is a ranking derived by laboratory standard test methodology of a material’s propensity to burn rapidly and spread flames. The higher the Flame Spread Index the more rapidly that flames will burn across the material surface. In general, the National Fire Protection Association defines three classes of materials depending on their Flame Spread Index:
Class | Flame Spread Index |
A | 0-25 |
B | 26-75 |
C | 76-200 |
The interior finish of all walls, columns, and partitions must not have a Flame Spread Index exceeding 200 except as otherwise specified herein. Ceiling interior finish must not have a Flame Spread Index exceeding 75. Walls adjacent to or enclosing a furnace or water heater and ceilings above them must have an interior finish with a Flame Spread Index not exceeding 25. Exposed interior finishes adjacent to the cooking range shall have a Flame Spread Index not exceeding 50. The HUD Code spells out the Flame Spread Index requirements for all elements of the home. Note that the terminology, Flame Spread Index and flame spread rating are used interchangeably.
Smoke alarm requirements.
- Each smoke alarm required must bear a label with evidence of conformance with HUD requirements
- Required smoke alarm locations. At least one smoke alarm must be installed in each of the following locations:
- To protect both the living area and kitchen space. Manufacturers are encouraged to locate the alarm in the living area remote from the kitchen and cooking appliances. A smoke alarm located within 20 feet horizontally of a cooking appliance must incorporate a temporary silencing feature or be of a photoelectric
- In each room designed for
- On the ceiling of the upper level near the top or above each stairway, other than a basement stairway in any multistory home. The alarm must be located so that smoke rising in the stairway cannot be prevented from reaching the alarm by an intervening door or obstruction
1.2.2.2.9 Structural design requirements.
General. Each manufactured home shall be designed and constructed as a completely integrated structure capable of sustaining the design load requirements of this standard, and shall be capable of transmitting these loads to stabilizing devices without exceeding the allowable stresses or deflections. Roof framing shall be securely fastened to wall framing, walls to floor, and the floor to the chassis.
Fastening of structural systems.
Roof framing must be securely fastened to wall framing, walls to floor structure, and floor structure to chassis, to secure and maintain continuity between the floor and chassis in order to resist wind overturning, uplift, and sliding, and to provide continuous load paths for these forces to the foundation or anchorage system. The number and type of fasteners used must be capable of transferring all forces between elements being joined.
Walls.
The walls shall be of sufficient strength to withstand the load requirements. The connections between the bearing walls, floor, and roof framework members shall be fabricated in such a manner as to provide support for the material used to enclose the manufactured home and to provide for transfer of all lateral and vertical loads to the floor and chassis.
Floors.
- Floor assemblies shall be designed in accordance with accepted engineering practice standards to support a minimum uniform live load of 40 lb/ft2 plus the dead load of the materials. In addition (but not simultaneously), floors shall be able to support a 200–pound concentrated load on a one-inch diameter disc at the most critical location with a maximum deflection not to exceed one-eighth inch relative to floor framing.
- Wood, wood fiber or plywood floors or subfloors in kitchens, bathrooms (including toilet compartments), laundry areas, water heater compartments, and any other areas subject to excessive moisture shall be moisture resistant or shall be made moisture resistant by sealing or by an overlay of nonabsorbent material applied with water-resistant adhesive.
Roofs.
- Roofs shall be of sufficient strength to withstand the load requirements. The connections between roof framework members and bearing walls shall be fabricated in such a manner to provide for the transfer of design vertical and horizontal loads to the bearing walls and to resist uplift forces.
- Roofing membranes shall be of sufficient rigidity to prevent deflection which would permit ponding of water or separation of seams due to wind, snow, ice, erection or transportation forces.
- Cutting of roof framework members for passage of electrical, plumbing or mechanical systems shall not be allowed except where substantiated by engineering
- All roof penetrations for electrical, plumbing or mechanical systems shall be properly flashed and sealed. In addition, where a metal roof membrane is penetrated, a wood backer shall be installed. The backer plate shall be not less than 5⁄16 inch plywood, with exterior glues, secured to the roof framing system beneath the metal
Windstorm protection.
Each manufactured home shall have provisions for support/anchoring or foundation systems that, when properly designed and installed, will resist overturning and lateral movement (sliding) of the manufactured home as imposed by the respective design loads.
Anchoring Systems.
- The provisions of this section shall be followed and the support and anchoring systems shall be designed by a Registered Professional Engineer or
- The manufacturer of each manufactured home is required to make provision for the support and anchoring systems but is not required to provide the anchoring equipment or stabilizing devices.
- For anchoring systems, the instructions provided by the Engineer or Architect shall indicate:
- The minimum anchor capacity required;
- That anchors should be certified by a professional engineer, architect, or a nationally recognized testing laboratory as to their resistance;
- That ground anchors should be embedded below the frost line and be at least 12 inches above the water table; and
- That ground anchors should be installed to their full depth, and stabilizer plates should be installed to provide added resistance to overturning or sliding
- Anchoring equipment should be certified by a registered professional engineer or architect to resist these specified forces in accordance with testing procedures in ASTM Standard
1.2.2.2.10 thermal protection
Ceiling vapor retarders.
- In Thermal Zones 2 and 3, ceilings must have a vapor retarder installed on the living space side of the roof cavity.
- For manufactured homes designed for Thermal Value Zone 1, the vapor retarder may be omitted.
Exterior wall vapor retarders.
- Exterior walls must have a vapor retarder installed on the living space side of the wall; or
- Unventilated wall cavities must have an external covering and/or sheathing that forms the pressure envelope. Formed exterior siding applied in sections with joints not caulked or sealed, are not considered to restrict water vapor transmission; or
Air infiltration.
The opaque envelope shall be designed and constructed to limit air infiltration to the living area of the home. Any design, material, method or combination that accomplishes this goal may be used. The goal of the infiltration control criteria is to reduce heat loss/heat gain due to infiltration as much as possible without impinging on health and comfort and within the limits of reasonable economics.
- Envelope penetrations. Plumbing, mechanical and electrical penetrations of the pressure envelope, and installations of window and door frames shall be constructed or treated to limit air infiltration. Penetrations of the pressure envelope made by electrical equipment, other than distribution panel boards and cable and conduit penetrations, are exempt from this requirement. Cable penetrations through outlet boxes are considered exempt.
- Joints between major envelope elements. Joints not designed to limit air infiltration between wall-to-wall, wall-to- ceiling and wall-to-floor connections shall be caulked or otherwise sealed. When walls are constructed to form a pressure envelope on the outside of the wall cavity, they are deemed to meet this requirement.
1.2.2.3 Plumbing Systems
1.2.2.3.1 General requirements
Any plumbing system installed in a manufactured home shall conform, at least, with the provisions of this subpart.
- General. The plumbing system shall be of durable material, free from defective workmanship, and so designed and constructed as to give satisfactory service for a reasonable life expectancy.
- Conservation. Water closets shall be selected and adjusted to use the minimum quantity of water consistent with proper performance and cleaning.
- Connection to drainage system. All plumbing, fixtures, drains, appurtenances, and appliances designed or used to receive or discharge liquid waste or sewage shall be connected to the manufactured home drainage system in a manner provided by this
- Workmanship. All design, construction, and workmanship shall be in conformance with accepted engineering practices and shall be of such character as to secure the results sought to be obtained by this standard.
- Components. Plumbing materials, devices, fixtures, fittings, equipment, appliances, appurtenance, and accessories intended for use in or attached to a manufactured home shall conform to applicable standards.
1.2.2.3.2 Water distribution systems.
- Supply piping. Piping systems shall be sized to provide an adequate quantity of water to each plumbing fixture at a flow rate sufficient to keep the fixture in a clean and sanitary condition without any danger of backflow or siphonage. The manufacturer shall include in his written installation instructions that the manufactured home has been designed for an inlet water pressure of 80 psi, and a statement that when the manufactured home is to be installed in areas where the water pressure exceeds 80 psi, a pressure reducing valve should be installed.
- Hot water supply. Each manufactured home equipped with a kitchen sink, and bathtub and/or shower shall be provided with a hot water supply system including a listed water
1.2.2.3.3 Drainage systems.
- Each fixture directly connected to the drainage system shall be installed with a water seal trap.
- The drainage system shall be designed to provide an adequate circulation of air in all piping with no danger of siphonage, aspiration, or forcing of trap seals under conditions of ordinary use.
- Materials
- Drainage piping shall be standard weight steel, wrought iron, brass, copper tube DWV, listed plastic, cast iron, or other listed or approved materials.
- Drainage fittings shall be recessed drainage pattern with smooth interior waterways of the same diameter as the piping and shall be of a material conforming to the type of piping used. Drainage fittings shall be designed to provide for a 1⁄4 inch per foot grade in horizontal piping.
1.2.2.3.4 Heating, Cooling, and Fuel Burning Systems
Heating, cooling and fuel burning appliances and systems in manufactured homes shall be free of defects, and shall conform to applicable standards in the following table unless otherwise specified in this standard.
1.2.2.3.5 Gas piping systems.
General. The requirements of this section shall govern the installation of all fuel gas piping attached to any manufactured home. The gas piping supply system shall be designed for a pressure not exceeding 14 inch water column (1⁄2 psi) and not less than 7 inch water column (1⁄4 psi). The manufacturer shall indicate in his written installation instructions the design pressure limitations for safe and effective operation of the gas piping system. None of the requirements listed in this section shall apply to the piping supplied as a part of an appliance. All exterior openings around piping, ducts, plenums or vents shall be sealed to resist the entrance of rodents.
Materials. All materials used for the installation, extension, alteration, or repair of any gas piping system shall be new and free from defects or internal obstructions. It shall not be permissible to repair defects in gas piping or fittings. Inferior or defective materials shall be removed and replaced with acceptable material. The system shall be made of materials having a melting point of not less than 1,450 F.
1.2.2.3.6 Heat producing appliances.
- Heat-producing appliances and vents, roof jacks and chimneys necessary for their installation in manufactured homes shall be listed or certified by a nationally recognized testing agency for use in manufactured
- A manufactured home shall be provided with a comfort heating
- When a manufactured home is manufactured to contain a heating appliance, the heating appliance shall be installed by the manufacturer of the manufactured home in compliance with applicable sections of this
- When a manufactured home is manufactured for field application of an external heating or combination heating/cooling appliance, preparation of the manufactured home for this external application shall comply
1.2.2.3.7 Exhaust duct system and provisions for the future installation of a clothes dryer.
All gas and electric clothes dryers shall be exhausted to the outside by a moisture-lint exhaust duct and termination fitting. When the clothes dryer is supplied by the manufacturer, the exhaust duct and termination fittings shall be completely installed by the manufacturer. However, if the exhaust duct system is subject to damage during transportation, it need not be completely installed at the factory when:
- The exhaust duct system is connected to the clothes dryer, and
- A moisture lint exhaust duct system is roughed in and installation instructions are provided.
- A clothes dryer moisture-lint exhaust duct shall not be connected to any other duct, vent or chimney.
- The exhaust duct shall not terminate beneath the manufactured
- Moisture-lint exhaust ducts shall not be connected with sheet metal screws or other fastening devices which extend into the interior of the
- Moisture-lint exhaust duct and termination fittings shall be installed in accordance with the appliance manufacturer’s printed
1.2.2.3.8 Appliances, cooling.
Every air conditioning unit or a combination air conditioning and heating unit shall be listed or certified by a nationally recognized testing agency for the application for which the unit is intended and installed in accordance with the terms of its listing.
1.2.2.3.9 Circulating air systems.
Supply ducts and any dampers contained therein shall be made from galvanized steel, tin- plated steel, or aluminum. A duct system integral with the structure shall be of durable construction that can be demonstrated to be equally resistant to fire and deterioration.
1.2.2.4 Electrical Systems
- The National Electrical Code, and specifically Article 550 of the National Electrical Code (NFPA No. 70–2005), plus the HUD Code address the electrical conductors and equipment installed within or on manufactured homes and the conductors that connect manufactured homes to a supply of
- The provisions of the HUD Code apply to manufactured homes intended for connection to a wiring system nominally rated 120/240 volts, 3-wire AC, with grounded
- All electrical materials, devices, appliances, fittings and other equipment shall be listed or labeled by a nationally recognized testing agency and shall be connected in an approved manner when in
- Aluminum conductors, aluminum alloy conductors, and aluminum core conductors such as copper clad aluminum; are not acceptable for use in branch circuit wiring in manufactured homes.
1.2.2.4.1 Power supply.
The power supply to the manufactured home shall be a feeder assembly consisting of not more than one listed 50 ampere manufactured home power supply cords, or a permanently installed circuit. A manufactured home that is factory-equipped with gas or oil-fired central heating equipment and cooking appliances shall be permitted to be provided with a listed manufactured home power-supply cord rated 40 amperes.
1.2.2.4.2 Disconnecting means and branch-circuit protective equipment.
- The branch-circuit equipment is permitted to be combined with the disconnecting means as a single assembly. Such a combination is permitted to be designated as a distribution panelboard. If a fused distribution panelboard is used, the maximum fuse size of the mains must be plainly marked with lettering at least 1⁄4-inch high and that is visible when fuses are changed.
- Plug fuses and fuseholders shall be tamper-resistant, Type ‘‘S,’’ enclosed in dead-front fuse panelboards. Electrical distribution panels containing circuit breakers shall also be dead-front type.
- A single disconnecting means shall be provided in each manufactured home consisting of a circuit breaker, or a switch and fuses and their accessories installed in a readily accessible location near the point of entrance of the supply cord or conductors into the manufactured home. The main circuit breakers or fuses shall be plainly marked ‘‘Main.’’ This equipment shall contain a solderless type of grounding connector or bar for the purposes of grounding with sufficient terminals for all grounding conductors. The neutral bar termination of the grounded circuit conductors shall be insulated.
1.2.2.4.3 Grounding.
- General. Grounding of both electrical and nonelectrical metal parts in a manufactured home shall be through connection to a grounding bus in the manufactured home distribution panelboard. The grounding bus shall be grounded through the green-colored conductor in the supply cord or the feeder wiring to the service ground in the service-entrance equipment located adjacent to the manufactured home location. Neither the frame of the manufactured home nor the frame of any appliance shall be connected to the neutral conductor in the manufactured home.
- Insulated neutral. The grounded circuit conductor (neutral) shall be insulated from the grounding conductors and from equipment enclosures and other grounded parts. The grounded (neutral) circuit terminals in the distribution panelboard and in ranges, clothes dryers, counter-mounted cooking units, and wall-mounted ovens shall be insulated from the equipment enclosure.
Chapter 1.3: The Florida Building Code – Advanced Fabricated Building
The Florida Building Code is patterned after the International Construction Code but has modifications, additions and deletions that are specific to Florida. The Florida Building replaced Florida’s patchwork of codes and regulations that were developed, amended, administered, and enforced by more than 400 local jurisdictions and state agencies with building code regulation responsibilities. The current Code is a single statewide code based on national model codes and consensus standards, amended for Florida specific needs for the design and construction of buildings. The Code is designed to make the local building process more efficient, increase accountability, bring new and safer products to the market, increase consumer confidence, and better protect the residents of Florida.
The base codes for the sixth Edition (2017) of the Florida Building Code Residential is the International Residential Code, 2015 edition; the National Electrical Code, 2014 edition; plus substantive criteria from the American Society of Heating, Refrigerating and Air-Conditioning Engineers’ (ASHRAE) Standard 90.1-2013. State and local codes adopted and incorporated into the code include the Florida Building Code, Accessibility, and special hurricane protection standards for the High-Velocity Hurricane Zone.
The Florida Building Code is adopted and updated with new editions every three years by the Florida Building Commission. It is amended annually to incorporate interpretations, clarifications and to update standards. Minimum requirements for permitting, plans review and inspections are established by the Code, and local jurisdictions may adopt additional administrative requirements that are more stringent.
The purpose of this module is to provide an understanding of the basic minimum home building requirements as outlined by the international building code, and the importance of adhering to minimum code requirements. The module includes the following sections:
- The permitting and inspection process
- Building planning and construction requirements
- The means of egress system
- Minimum mechanical and plumbing requirements
1.3.1 Permits and Inspections
1.3.3.1 Permit Application:
The code-enforcement process is normally initiated by an application for a permit to construct or remodel a building. In the state of Florida, a permit is required for any owner or authorized agent who intends to construct, enlarge, alter, repair, move, demolish, or change the occupancy of a building or structure, or to erect, install, enlarge, alter, repair, remove, convert or replace any required impact-resistant coverings, electrical, gas, mechanical or plumbing system, the installation of which is regulated by code, or to cause any such work to be done.
To obtain a building permit one must first make an application with the building official, by filing an application in writing on a form furnished by the building department. Each application will have the date of application, and the building code version that is in effect as of that date. The building official is responsible for processing applications and issuing permits.
1.3.1.2 Application Requirements:
An application for a permit is good for 180 days after the date of filing. The building official can grant one or more extensions for no more than 90 days each as long as the extension is requested in writing and has justifiable cause. As a condition to receiving a permit, the applicant must show proof that workers’ compensation coverage has been provided for employees and contractors have the required licenses.
Additionally, the Florida Building Code requires that the permit applicant can adequately demonstrate that construction for the proposed project complies with the code. This is primarily accomplished by submitting construction documents for the project along with the application for permit.
Construction Documents:
Within the construction documents each official is looking for sufficient clarity and detail of the location, nature and extent of the work proposed, as well as conformance to the provisions of the building code. Specifically, construction documents need to be prepared by a design professional and affixed with their seal as required by Florida law; and they should be dimensioned and drawn upon suitable material.
Review of construction documents by the building official shall include the following minimum criteria and documents: a floor plan; site plan; foundation plan; floor and roof framing plan or truss layout; and all exterior elevations.
Once the construction documents are reviewed, approved and stamped as “Reviewed for Code Compliance”, one set is kept by the building official, the other set is returned to the applicant to be kept at the job site, and the building permit is issued. Even after the permit is issued, the building official can inspect the approved plans for errors or code violations and require corrections as such. Work done should be in accordance with the approved construction drawings, any changes made during construction which deviate from the approved drawings, need to be resubmitted for approval as an amended set of documents.
1.3.1.5 Permit Longevity:
A permit becomes null and void after 6 months if the work has not been started or if the work was started but is suspended or abandoned for a period of 6 months. Lack of at least one approved inspection within 180 days of permit issuance will serve as a validation that the job has been suspended or abandoned. Once the permit has been issued, a copy must be kept on the jobsite until completion of the project. The building permit and inspection card must be in plain view on
1.3.1.5 Inspections:
Work that requires a permit is also subject to inspections by the building official. A list of required inspections is located on the bottom of the permit placard. Instructions on scheduling inspections are provided when the permit is issued. It is the applicant’s responsibility to ensure that all required inspections are made prior to proceeding with work beyond each successive point.
Upon notification from the permit holder, the building official makes these inspections. However, the timing and sequencing of when these inspections occur and what elements are inspected is up to the building official.
If the work passes inspection, then the official will release that portion of the construction, if not, the permit holder will be informed of violations which must be corrected to comply with applicable codes and to proceed with work.
For new dwellings, once all fees are paid and all required inspections are passed and completed, the applicant will be issued a Certificate of Occupancy (commonly called a CO).
1.3.2 Building Planning and Construction
1.3.2.1 Building Planning
It is important for design and construction professionals to adhere to basic standards for building a home, starting with the structural frame. Strengthening the structural frame of a house includes creating a “complete load path” within the home, which is a method of construction that uses a system of wood, metal connectors, fasteners (like nails and screws) and shear walls to connect the structural frame of the house together. A complete load path is like a chain that ties the house together from the roof to the foundation. A home is more likely to withstand a seismic or high wind event and stay intact when all parts of the house – roof, walls, floors and foundation – are connected together securely.
The Florida Building Code requires all buildings and structures, and all parts thereof, to be constructed in a way that safely support all loads, including dead loads, live loads, roof loads, flood loads and wind loads. And a continuous load path between foundations, walls, & roofs must be provided. (FBC- Residential R501.2 Requirements and R601.2 Requirements)
1.3.2.2 Florida Building Code Loads
Dead Loads – The weight of materials of construction incorporated into the building, including walls, floors, roofs, ceilings, stairways, finishes, cladding and other architectural or structural items, and the weight of fixed service equipment such as cranes, plumbing stacks and risers, electrical feeders, heating, ventilating and air-conditioning systems and fire sprinkler systems.
Live Loads – Those loads produced by the use and occupancy of the building or other structure and do not include construction or environmental loads such as wind load, snow load, rain load, earthquake load, flood load or dead load.
Live Loads (Roof) – Those loads produced (1) during maintenance by workers, equipment and materials; and (2) during the life of the structure by movable objects such as planters and by people.
Nominal Loads – The magnitudes of other design loads including soil, wind, snow, rain, flood and earthquake.
Constructing homes in accordance with the provisions of the code should result in a system that provides a complete load path, which will transfer of all loads from their point of origin through the load-resisting elements to the foundation.
1.3.2.3 Exterior Walls
Exterior walls of the house must create a weather-resistant exterior wall envelope and include corrosion-resistant flashing. One purpose of the wall envelope is to prevent the accumulation of water within the wall assembly. One way it can be accomplished is by using weather- resistant sheathing paper such as No. 15 asphalt felt or other approved water-resistive barrier over studs or sheathing of all exterior walls. Concrete and masonry walls properly constructed and flashed in accordance with code specifications do not require a weather-resistant exterior wall envelope.
1.3.2.4 Interior Wall Coverings
Interior coverings and finishes must be installed as required by the code which outlines the minimum thickness for plaster, gypsum plaster proportions, maximum volume aggregate per volume of cementitious material for Portland cement plaster, and minimum thickness for gypsum drywall. Interior finishes and materials must comply with the flame spread and smoke- development requirements which state that wall and ceiling finishes shall have a flame-spread classification of no greater than 200 and a smoke-developed index of no greater than 450.
1.3.2.5 Minimum Room Dimensions
The total area of a residence is generally not regulated by code; however, a structure more than three stories in height would have to meet the provisions of the Florida Building Code- Building, rather than the Florida Residential Code. Only homes three stories or less, with a separate means of egress for accessory structures (like a garage or carport) fall under the provisions of the Residential Code. The Code does, however, have several minimum requirements that apply to residences. Residential space standards provide specifications for the internal floor area of dwellings and rooms.
Every dwelling must have at least one habitable room that has a minimum of 120 square feet of gross floor area. In most cases, this larger room is designed as the living area in a home. Other habitable rooms must have a floor area of at least 70 square feet and cannot have any one wall less than 7 feet in length.
The kitchen, however, though still considered a habitable room, can be a little smaller, but not less than 50 square feet in gross floor area. A habitable room is defined as all rooms used for living, dining, sleeping and cooking purposes. Bathrooms, closets, halls, and utility spaces are not considered habitable rooms.
When figuring the square footage of a room, any portions of the room with a sloped ceiling measuring less than 5 feet or a furred ceiling measuring less than 7 feet from the finished floor to the finished ceiling are not included as habitable space for the room.
The home designer has the flexibility to distribute the total amount of space among the rooms as they wish, as long as the combined spaces and the individual rooms meet minimum code requirements.
1.3.2.6 Ceiling Heights and Insulation
Habitable space, hallways, bathrooms, toilet rooms, laundry rooms, and portions of basements containing these spaces cannot have a ceiling less than 7 feet.
There are some exceptions to that provision:
- Rooms with sloped ceilings need to have a ceiling height of at least 7 feet in no less than 50% of the required floor area of the room, and no portion of the required floor area can have a ceiling height of less than 5
- The ceiling in a bathroom must be at least 6 feet 8 inches over bathroom fixtures as well as at the center of the clearance area in front of the fixtures, which ranges from 21” to 24” depending on the fixture type. In a shower or tub area with a showerhead, there are minimum space requirements, which include a 30 inch x 30 inch area at the showerhead and a ceiling height of no less than 6 feet 8 inches
The code further specifies that ceilings in basements without habitable space, hallways, bathrooms, toilet rooms and laundry rooms can be as low as 6 feet 8 inches in height, and beams, ducts, or other obstructions may project to within 6 feet 4 inches of the finished floor.
Often people renovate an attic to increase their home’s resale value but spaces with a ceiling height of less than 7 feet don’t contribute to a home’s square footage in terms of appraisal.
Insulation materials must have a flame spread index not to exceed 25 with an accompanying smoke-developed index not to exceed 450.
1.3.2.7 Garages
The relationship between the garage and the habitable spaces of the home, require special attention and detailing. In order to prevent carbon monoxide or fumes from other hazardous materials stored in the garage from entering the house, openings from the garage leading directly into a bedroom space are not permitted by the Florida Building Code. Other openings between the garage and residence shall be equipped with either a solid wood door, solid, or honeycomb core steel door, none of which can be less than 1 3/8” thick, or you can use a 20- minute fire-rated door. The garage must be separated from the home interior and attic area by a minimum of ½” thick gypsum board applied to the garage side.
Garages situated beneath habitable rooms require a separation of at least 5/8” Type X gypsum board or equivalent.
There are two types of gypsum wallboard, regular and type X. Type X wallboard is formulated by adding noncombustible fibers to the gypsum. These fibers help maintain the integrity of the core as shrinkage occurs, providing greater resistance to heat transfer during fire exposure. Type X gypsum is typically required to achieve required fire resistance ratings.
1.3.2.8 Detached Garages and Carports:
Detached garages on the same lot as a house but located less than 3 feet away from it, require at least ½” gypsum board applied to the interior side of exterior wall that faces the home, this extra protection is not required on the garage walls perpendicular to the house.
There should be no opening for air duct systems into the garage, such as returns or vents. These might actually “fuel” a fire. Any ducts passing through the garage or penetrating the separating wall or ceiling are required to be a minimum of 26-gauge sheet steel, 1 inch minimum rigid nonmetallic Class 0 or Class 1 duct board, or other approved material.
Garage floors are required to be of an approved noncombustible material, and should be sloped to facilitate the movement of liquids either to a drain or toward the main vehicle entry.
Carports need to be open on at least two sides, and have approved noncombustible floor material. Ground level carports can have asphalt surfaces. If a carport is not open on at least two sides, it’s considered to be a garage according to code, and must meet the minimum provisions for garages.
1.3.3 Means of Egress and Emergency Openings
1.3.3.1 Means of Egress
The Florida Building Code defines “means of egress” as a travel system that provides a continuous, unobstructed and undiminished path from an occupied point in a building or structure to a public way.
A means of egress is required by the building code to provide a point of emergency escape and rescue. The term “egress” is used in most building codes to specify areas of access or exits, typically doors, windows, and hallways. The phrase “means of egress” refers to the ability to exit the structure, primarily in the event of an emergency, such as a fire.
A means of egress comprised of three distinct parts: the path of travel to an exit, the exit itself, and the exit discharge (the path to a safe area outside).
Here are definitions of these terms, as well as some other important definitions for understanding the means of egress system.
1.3.3.2 Definition of Means of Egress Terms
- EXIT ACCESS: The portion of a means of egress system that leads you from occupied space to an exit. It includes any room or space occupied by a person and any doorway, aisle, corridor, stair, or ramp traveled on the way to the
- EXIT: The portion of a means of egress system that is between the exit access and the exit discharge or public way. This area is separated from other interior spaces by fire- resistance-rated construction and opening protectives as required. It can be very basic, such as an exterior door, or it can include enclosed stairwells and ramps. In some special cases, it can include exit corridors or passageways. The building materials used for an exit require higher fire ratings than the materials for exit
- EXIT DISCHARGE: The portion of a means of egress system between the termination of an exit and the public way. It can be inside a building such as the main lobby, or outside a building such as an exterior vestibule, courtyard, patio, small alley, or other safe passageway.
- PUBLIC WAY: An area exposed to open air that leads to a street permanently appropriated for the public and public use. This area can be a street, alley or other land parcel, but by definition has a minimal clear width and height of 10’. What distinguishes a public way from an exit discharge is its size. For example, any alley can be an exit discharge, but if it is 10 feet wide and 10 feet high and it leads to a public street, it is a public
1.3.3.3 Exit Doors
The number of exits required for a structure is based on its intended use and occupancy. Because the normal number of occupants in a residence is less than in a similarly-sized non- residential space, determining a safe level of means of egress is not as complicated.
The Building Code requires all dwellings to have at least one egress door that has direct access from a continuous unobstructed path of travel from all portions of the dwelling to the exterior, without requiring travel through a garage.
The required exit door must be side-hinged and cannot be less than 32” wide by 6’ 6” high. Other doors to the exterior are allowed to have smaller widths.
Each exterior door is required to have a floor or landing on each side of the door except when a stairway with two or fewer risers is located on the exterior side of the door.
The width of the landing should not be less than the width of the door and should have a minimum dimension of 36 inches measured in the direction of travel.
1.3.3.4 Emergency Exits
Basements and each sleeping room need to have at least one operable emergency escape and rescue opening with a minimum clear opening of 5.7 square feet, which opens directly into a public street, public alley, yard, or court.
Emergency escape openings can have a minimum net clear opening height of 24 inches or a minimum width of 20 inches, and must have sill height within 44 inches of the floor.
Emergency escape openings must be operable from the inside of the room without the use of keys or tools or special knowledge. This gives occupants in each sleeping area a method of escape in case of a fire.
1.3.3.5 Windows and Glazing
The light and ventilation requirements of the building code have a major effect on window type, size and placement. Window glass, called glazing, has come a long way since the days when windows had only a single pane of glass. Today’s energy-efficient windows come with glazing “systems” that incorporate multiple panes of glass, gas fillings, and high-tech, heat- sensitive coatings. These new and improved windows may cost more but they pay off in increased comfort and reduced energy costs.
Window Glazing – Ratings:
Window glazing is rated on three criteria: how well it insulates, how much light it lets through, and how effectively it blocks heat from the sun. By taking these values into consideration, you can effectively choose the best windows for the climatic conditions of the property, and even tailor individual windows to best suit specific rooms in the house.
Window Glazing- Hazardous Locations:
The Building Code requires each pane of glass to bear the manufacturer’s label, indicating the type and thickness of glass or glazing material. This safety glazing label has to be acid etched, sandblasted, ceramic-fired, embossed mark, or once applied unable to be removed without being destroyed.
The following are considered hazardous locations for glazing and require special attention or detailing:
- Glazing in swinging doors and storm
- Glazing in sliding and bifold closet
- Glazing in unframed swinging
- Glazing in doors, enclosures, walls and fences enclosing hot tubs, saunas, bathtubs, and showers.
- Glazing in an individual fixed or operable panel next to a door where the nearest vertical edge is within 24 inch arc of the door and the bottom edge is less than 60 inches above any walking
- Glazing in
- Glazing next to stairways, landings and ramps within 36 inches of walking
Window Glazing- Habitable Rooms:
The FBC states that all livable rooms need to have a combined glazing area of not less than 8 percent of the floor area of that room. Natural ventilation should be provided through windows, doors, louvers, or other approved openings to the outside air. These openings need to be readily accessible and controllable by the building occupants. The minimum openable area to the outdoors should be 4 percent of the floor area being ventilated.
Window Glazing- Bathrooms:
Bathrooms have different requirements: There should not be less than 3 square feet of combined glazing area in windows in bathrooms, water closets and similar rooms, half of which is readily controllable.
You can avoid this requirement by providing artificial light and a mechanical ventilation system. The minimum ventilation rates are 50 cubic feet per minute for intermittent ventilation or 20 CFM for continuous ventilation (which is a constant and controlled level of ventilation). Don’t forget ventilated air must always be exhausted directly to the outside.
Window Glazing- Opening Details:
Required glazed openings need to open directly onto a street, public alley, yard, or court located on the same lot as the building.
They can open onto a roofed porch as long as the longer side of the porch is at least 65 percent open and unobstructed and there’s a ceiling height of not less than 7 feet.
Windows are permitted to open into sunroom additions or patio covers that look onto a street, yard or court as long as more than 40 percent of the exterior sunroom walls are open, or are enclosed only by insect screening and if the ceiling height is not less than 7 feet.
The alternative to providing openable windows is to provide mechanical ventilation. Mechanical heating and cooling will be discussed in more depth in Section 5 of this module.
1.3.3.6 Exit Access- Stairways
Stairs can often dictate the layout of an entire structure. Because of their importance in the design process, stairs must be considered early on. The location of railings and balusters, the width and depth of treads, and the height of risers can all affect a stairway’s ease and safety of use, both in terms of everyday use and as part of a means of egress system. Therefore, these dimensions are regulated by most building codes.
The Florida Building Code requires that exterior landings, decks, balconies, stairs and similar facilities, must be positively anchored to the primary structure to resist vertical and lateral forces or shall be designed to be self-supporting. Attachment cannot be completed with nails, such as toenails, that are subject to withdrawal.
Treads, Risers & Landings:
The maximum height of a stair riser is 7 ¾ inches. Within any flight of stairs, the actual measurement cannot be more than 3/8” difference between the greatest riser height and the smallest
The minimum tread depth cannot be less than 10 inches. As with the stair risers, to achieve uniformity of motion, the actual measurement of the tread depth cannot be more than 3/8” difference between the greatest depth and the smallest.
Headroom must also be considered. In all parts of the stairway the minimum headroom is 6 feet 8 inches. This is measured vertically from the sloped plane created by adjoining tread nosings as well as from the finished floor of the landing or platform.
Allowing for this required headroom influences wall placement on an upper floor over the stairwell. Stairways can’t have less than 36 inches clear width between the height of the handrail and the headroom.
Landings should be provided at the top and bottom of each stairway. No vertical rise of more than 12’ is allowed between floor levels or landings. Additionally, the width of the landing can’t be smaller than the stairway it connects to, and every landing needs a minimum dimension of 36 inches measured in the direction of travel. The pitch of treads and landings cannot exceed 2%.
Stairway Lighting:
Lighting is an important element in stairway design. It does not need to be especially bright, but the risers and treads should have sufficient light to ensure that they can be distinguished. All interior and exterior stairways, including landings and treads, must be provided with a means of illumination.
Interior stairways must include an artificial light source located in the immediate vicinity of each landing, capable of illuminating the steps a minimum of 1 foot-candle. Exterior stairways need an artificial light source located at the top landing. Interior stairways with 6 or more risers need to include a light switch on the wall at both the top and bottom of the stairs. Exterior stairways need a light switch that can be controlled from inside the house. However, if the stairway lighting is continuously illuminated or automatically controlled, these switch requirements are not necessary.
Handrail Requirements:
Guards and handrails are needed by a variety of people for very different purposes. For example, they protect people from falling off the edge of the stair tread or landing but also help people keep their balance and provide leverage when ascending/descending stairs.
The maximum projection of the handrails off the wall is 4.5” on either side of the stairway. The space between the wall and the inner edge of the handrail can’t be less than 1- ½”. For a stairway with a handrail on only one side there must be a minimum clear width of 31.5 inches at the height of and below the handrail projection. For a stairway with handrails on both sides, this clear width can’t be less than 27”.
Any stairway with 4 or more risers has to have a handrail on at least one side. The height of which should be between 34 and 38” from the sloped plane created by adjoining adjacent nosings.
Handrails should be continuous for the full length of the flight and the ends should be either returned or terminated in newel posts or safety terminals.
The outside diameter of circular-shaped handrails must be between 1 ¼” and 2 inches. Handrails with a non-circular cross section need a perimeter dimension of 4 to 6 ¼ inches.
Ramps & Guards:
A ramp is an inclined plane that functions with or instead of stairs. Ramps permit wheelchair users, as well as people pushing strollers, carts, or other wheeled objects, to traverse an elevation change.
The maximum slope of a ramp is 8.3 percent. A 3’ by 3’ landing is required at the top and bottom of the ramp, where ramps change directions, and where a door opens onto a ramp.
Guards are building components located at the open side of an elevated walking surface, its purpose is to minimize the possibility of a fall to the lower level.
Porches, balconies, ramps, or raised floor surfaces that are 30 inches or more above the floor below require 36” minimum guards. Stairways that rise 30” or more above the floor below must have 34” minimum guards on the open side of the stair. Screened porches, sunrooms and decks 30” above the ground or floor elevation also required guards.
Where guards are required they should be equipped with intermittent rails or ornamental closures which do not allow passage of a sphere 4 inches or more in diameter.
1.3.3.7 Smoke Alarms
A means of egress system is designed to provide safe and continuous passage of emergency escape out of a structure and into public space. In addition to this travel corridor in an emergency situation there are other building components which contribute to safe escape. Such items include effective lighting, properly placed exit signs, and operable smoke alarms. Of course for residential design the latter is of the upmost importance.
According to the Residential Florida Building Code, smoke alarms should be installed in each bedroom, outside each separate sleeping area in the immediate vicinity of the bedrooms, and on each additional story of the dwelling, including basements but not including crawl spaces and uninhabitable attics.
If the dwelling is split level without an intervening door between adjacent levels, a smoke alarm on the upper level will suffice for the adjacent lower level, as long as it is less than a full story difference. To prevent false alarms, when locating fire alarms, be sure to avoid close proximity to the kitchen or fireplace.
All smoke alarms need to be listed in accordance with UL 217, a standard for electrically operated single and double station fire alarms intended for residential applications. Smoke alarms should be installed in accordance with the household fire warning equipment provisions of the National Fire Protection Association, NFPA 72.
Additionally, smoke alarms must be installed so as to receive primary power from the building’s electrical wiring, but should also contain a battery-powered back up system; and if more than one alarm is required they should be interconnected so that if one alarm activates, all the alarms in the dwelling unit will sound.
1.3.4 Plumbing and Mechanical
1.3.4.1 Sanitation
For sanitation purposes, the minimum plumbing requirements for a residence include providing a kitchen sink, toilet, lavatory and a bathtub or shower.
All plumbing fixtures need to be connected to an approved water supply and a sanitary sewer or an approved private sewage disposal system. Additionally, bathroom, kitchen and laundry outlets must be supplied with hot and cold water.
Hot water shall be supplied by an approved automatic water heater or other type of domestic water-heating system sufficient to supply hot water to fixtures and appliances in spaces intended for bathing, washing or cooking.
Storage tanks for hot water heaters must be constructed of or lined with noncorrosive metal. And need to be connected and located where they can be maintained, serviced, and replaced.
Ideally, hot water heaters should be installed in a room with a floor drain, and should be equipped with a shut-off valve, to minimize damage in the event of a leak.
1.3.4.2 Bathrooms Requirements:
All bathroom fixtures shall be spaced according to Figure R307.1. Important dimensions are:
- The centerline of the toilet or bidet must be at least 15” from a wall or other obstruction, and needs 21” of forward
- Sinks are shown in 4 different configurations- in all cases the sink should be 4” off the wall and have 21” of forward clearance. Double sinks should be separated by a minimum space of 4”. A sink next to a toilet also needs 4” separation space, but a sink next to a tub needs only 2” of
The minimum dimensions allowed for a shower is 30” x 30” (that’s 900 square inches), and there should be a minimum of 24” forward clearance at all shower entrances. Plumbing fixtures, faucets, and fittings must have smooth impervious surfaces, free of defects, and constructed of approved materials. Fixtures must be provided with a supply of potable water adequate to flush the fixture and maintain a clean and sanitary condition, without danger of backflow or cross connection.
FIGURE R307.1 MINIMUM FIXTURE CLEARANCES
Shower Specifications:
In addition to the minimum area of interior space required for showers by Figure R307.1, there must also be 70” of minimum height provided above the shower drain.
Shower openings must have a clear and unobstructed finished width of not less than 22”, and hinged shower doors, if used, must open outward.
Shower valves and shower/tub combination valves must be equipped with pressure-balance controls, thermostatic mixing, or a combination of the two. They must also include a high limit stop, set to limit the water temperature to 120 degrees maximum.
Walls and floors in shower and tub spaces must be of a nonabsorbent surface material that extends at least 6 feet above the floor. Such material cannot be water-resistant gypsum board. Although water-resistant gypsum board is permitted for use as a backer for tiles or wall panels in showers and tubs, it cannot be installed over the vapor retarder, as this would create a waterproof membrane on both sides of the gypsum board, trapping moisture and ultimately causing it to fail. Ideally, gypsum board should not be used in wet areas.
1.3.4.3 Mechanical Ventilation Systems
Mechanical ventilation systems circulate fresh air using ducts and fans. Ventilation systems significantly improve a home’s air quality by removing allergens, pollutants and moisture that can cause mold problems. They can provide proper fresh air flow with appropriate locations for intake and exhaust and can also provide filtration, dehumidification and conditioning of the outside air as it enters. Without ventilation moisture, odors, and other pollutants can build up inside a home.
1.3.4.4 Venting:
Ducts are used in heating, ventilation, and air conditioning systems, to deliver and remove air. Air ducts are one method of ensuring acceptable indoor air quality, as well as, thermal comfort. Outside air intake openings cannot be located within 10 feet of vents, chimneys, plumbing vents, streets, alleys, parking lots, or loading docks, as these are considered sources of hazardous or noxious contaminants. Exhaust from bathrooms and kitchens are not considered noxious or hazardous.
If a source of contaminant is located within 10 feet of intake openings the opening needs to be located at least 2 feet below the contaminant source. This will help prevent contaminants from being introduced into the ventilation system or exhaust from entering into occupied areas or into other buildings.
Exhaust openings located outdoors must not be directed on to walkways or create a nuisance by its location. Additionally, exhaust and intake openings that terminate outdoors should be protected from the weather with corrosion-resistant screens, louvers, or grilles with openings ¼” to ½” in any dimension.
1.3.4.5 Bathroom Exhaust:
Exhaust air from bathrooms and toilet rooms should not be recirculated within a residence or to another dwelling unit, but should instead be exhausted directly to the outdoors. This prevents the flow of contaminants from one unit to another.
Bathroom fans should not be vented into an attic or crawl space because it can cause moisture build-up, discoloration, and rot in the rafters. The humidity can damage the structure and insulation.
Exhaust fans should be located in or near the shower or tub, and in an enclosed water closet. Keep exhaust points opposite the supply air source to ensure that the fresh air is drawn through the room.
Bathroom doors should not be sealed too tightly at the bottom in order to allow “makeup air” to enter the room when the door is closed.
Ventilation fans in bathrooms should have the capacity to exhaust a minimum of 50 cubic feet per minute intermittently or 20 cubic feet per minute continuously.
1.3.4.6 Laundry Exhaust:
Clothes dryers emit high levels of moisture, which could lead to mold growth and building deterioration if vented to the indoors. Therefore, clothes dryers must be exhausted in accordance with the manufacturer’s instructions, be independent of all other systems, and convey any moisture and combustion products to the outside.
The exhaust should be terminated to the outside in accordance with the manufacturer’s installation instructions. In the absence of installation instructions, the exhaust duct cannot terminate less than 3 feet in any direction from openings into house. Screens should not be installed at the duct termination, but should instead be equipped with a backdraft damper, which is a damper with blades actuated by gravity, permitting air to pass through them in one direction only
1.3.4.7 Kitchen Specifications:
According to the Florida Building Code, a range hood is not required in a kitchen, unless an open-top broiler is installed. Domestic open-top broiler units must include a 28 gage metal exhaust hood. It should be installed with ¼ inch between the hood and the underside of combustible materials or cabinets. The cooking surface of the broiler unit needs a clearance of at least 24” from combustible materials or cabinets. The exhaust hood needs to be at least as wide as the broiler unit and extend over the entire unit. Additionally, it must exhaust to the outside and have a backdraft damper or other means to control infiltration and exfiltration when not in use. The ventilation rate required for kitchen exhaust is 100 cubic feet per minute intermittently or 25 cubic feet per minute continuously. All household cooking appliances must be listed, labeled and installed in accordance with the manufacturer’s installation instructions.
1.3.4.8 Fuel-fired appliances:
Gas-fired appliances such as stoves, ranges, heaters and lamps require special consideration. A supply of air for fuel combustion and ventilation of the space in which the appliance is installed must be provided. Failure to supply adequate air can result in improper draft, soot production, increased carbon monoxide production, and risk of fire or explosion; failure to vent properly can cause build-up of carbon monoxide, high temperatures, and increased risk of fire.
Fuel-fired appliances may not be located in bedrooms, bathrooms, or storage closets. They can however, be located in spaces that can only be accessed from and open into such rooms, but only if all combustion air is obtained from the outdoors through permanent openings with a solid weather-stripped door and self-closing device. This is to avoid build-up of combustion gases, or the depletion of oxygen levels. Asphyxiation can result from inadequate combustion air.
1.3.6 Protection against Termites
Before March 1st, 2002, there was no standard control for protection against termites, and building codes did not require contractors or pest control operators to prevent termite infestations. As a result, termite damage was at record levels. The effect of implementing requirements as per the Florida Building Code is now making builders more responsible for termite protection.
The Florida Residential Code states that termite protection must be provided by registered termiticides, including soil applied pesticides, baiting systems and pesticides applied to wood, or other approved methods of termite protection labeled for use as a preventative treatment to new construction.
A Certificate of Compliance will be issued by the licensed pest control company to the building department upon completion of the application of the termite protective treatment. It will state that the building has received complete treatment for the prevention of subterranean termites in accordance with the Florida Department of Agriculture and Consumer Services.
Chapter 1.4: Florida Energy Code (2017) Energy Conservation – Advanced Fabricated Building
For modular construction in Florida, the Florida Building Code regulates the quality, health, and safety of home construction by defining the types of materials and methods that are to be used in all aspects of construction. In addition to the Florida Building Code, modular homes must comply with the Florida Building Code (2017) Energy Conservation for homes which defines the requirements for the thermal performance of the building envelope (including walls, ceilings, roofs, windows, doors, and insulation); of heating, cooling, and ventilation (HVAC) system; and of electrical components such as the lighting system. The following sections provide information about some of the key requirements of the Florida Building Code (2017) Energy Conservation for homes.
1.4.1 Definition, Abbreviations, and Nomenclature
°F: degrees Fahrenheit
°C: degree Centigrade
Note that °C = (°F – 32) x 0.556. Thus 32°F = 0°C and 68 °F = 20°C
Building thermal envelope: the barrier between the interior and exterior of the home, it includes the walls, ceiling, roof , attic, windows, doors, and insulation. This barrier must be designed to minimize heat flow between the home interior and the outside.
Climate Zone: an area of a country with similar climate conditions. The US has 8 Climate Zones, seven of which are shown in Figure 1.8, with Zone 8 being in Alaska. Note that Florida has two Climate Zones, Zone 1 and Zone 2. Zone 1 is quite small and covers Miami-Dade and Broward counties. The remainder of Florida falls into Zone 2, along with much of the southeast Gulf coast.
Figure 1.8 Climate Zone map of the U.S. excluding Alaska which is in Climate Zone 8
fenestration: the glass components of a building’s façade including windows, glass doors, and storefronts.
glazing: another name for fenestration, that is, the glass components of the building envelope. IC-rated: recessed lighting with this rating can be safely installed in a ceiling and surrounded by insulation. Non-IC rated lighting means the recessed light cannot be installed surrounded by insulation and must have at least a 3 inch air gap around it. See Figure 1.9.
Figure 1.9 A properly installed IC-rated recessed or ‘can’ light which can be safely covered with insulation.
radiant barrier: a foil material used to reflect solar radiation, usually installed on the interior surface of the roof. Radiant barriers can greatly reduce heat being transferred into the house, thus reducing the cost of air-conditioning.
R-value: the thermal resistance of a material in units of hours × ft2 × °F/Btu. The higher the R- value, the lower the amount of heat that can flow through the material per hour. In most of Florida, outside of Miami-Dade and Broward counties, walls must have an R-value of 13 or more and ceilings have an R-value of 30 or higher. This is more compactly stated as walls must have a minimum R-13 and ceilings must have a minimum R-30 performance (see Figure 1.10). In terms of heat transfer, windows are the weak link in the building façade because they generally have fairly low R-values. For example, a window that meets the requirements of the Florida Energy Code will have a R-value of just 1. As a result, the tradeoff between having more or less glass in the home has to be assessed because more glass provides additional daylighting, reducing lighting costs. On the other hand, more glass also means more heat being transferred into the home by both conduction and radiation, increasing the cost of air-conditioning.
Figure 1.10 The picture above depicts how many inches of various materials are needed to produce a R-22 value. It is clear that insulating materials like cellulose fiber and fiberglass have much higher thermal resistance than wood or brick. (Source: Penn State University)
Solar Heat Gain Coefficient (SHGC): the ratio of solar radiation passing through a window to the total solar radiation striking the window. It is a measure of how much heat energy is passing through glass. SHGC has a minimum value of 0 and a maximum value of 1. A low SHGC, closer to 0, means that most of the heat energy of solar radiation is reflected back to the exterior rather than being transmitted inside (see Figure 1.11).
Figure 1.11 This window has a SHGC of 0.39 which means that it allows just 39% of the heat portion of the solar spectrum to pass through the glass into the interior of the home. VT stands for visible transmittance as is described below. (Source: www.oocities.org)
U-factor: a measure of the resistance to heat transfer through an assembly. It is roughly the inverse of the R-value, meaning that the lower the U-value, the higher the resistance to heat flow. This is just the opposite of the R-value because the higher the R-value the greater the resistance to heat flow.
In general: U = 1/R where R is the sum of the thermal resistance of the components of, for example a wall, which has an outer surface and an inner surface, that together sandwich the insulation, a moisture barrier, and the wall structure together in a single unit. It also includes the effects of air movement inside and outside the surface which can contribute to heat transfer.
Heat Flow = U-Factor x Surface Area x temperature difference between the two surfaces
or
Q (heat flow) = U x A x ΔT
Figure 1.12 Windows carry a National Fenestration Research Council (NFRC) label that indicates the U-Factor for the window assembly as a whole and also provides additional information about the window performance. In this case the U-Factor is 0.35 and the Solar Heat Coefficient is 0.32. (Source: Penn State University)
Visible Transmittance (VT): the fraction of the visible light spectrum that passes through the glass. Generally, windows with a lower SHGC will have a lower VT because the films in the low SHGC glass that block solar heat from passing through the window also prevent some visible light from being transmitted.
1.4.2 Interior design conditions.
The interior design conditions are stated in the Energy Code because this information is needed to size air-conditioning and heating equipment by means of load calculations The interior design temperatures used for heating and cooling load calculations shall be a maximum of 72°F (22°C) for heating and minimum of 75°F (24°C) for cooling.
1.4.3 Energy performance level (EPL) display card (Mandatory).
The building official shall require that an energy performance level (EPL) display card be completed and certified by the builder to be accurate and correct before final approval of the building for occupancy. Florida law requires the EPL display card to be included as an addendum to each sales contract for both presold and non-presold residential buildings. The EPL display card contains information indicating the energy performance level and efficiencies of components installed in a dwelling unit.
1.4.4 Requirements for the Building Thermal Envelope
1.4.4.1 Insulation and fenestration
The building thermal envelope shall meet the insulation requirements of Table R402.1.1. Note that Climate Zone 1 refers to Dade and Broward counties and Climate Zone 2 includes all other Florida counties
TABLE R402.1.1 INSULATION AND FENESTRATION REQUIREMENTS BY COMPONENT
CLIMATE ZONE | FENESTRATION U– FACTOR | SKYLIGHT U– FACTOR | GLAZED FENESTRATION SHGC | CEILING R– VALUE | WOOD FRAME WALL R-VALUE | MASS WALL R– VALUE | FLOOR R– VALUE | BASEMENT Wall R-VALUE | SLAB R-VALUE & DEPTH | CRAWL SPACE WALL R-VALUE |
1 | .65 | 0.75 | 0.25 | 30 | 13 | 3/4 | 13 | 0 | 0 | 0 |
2 | 0.40 | 0.65 | 0.25 | 38 | 13 | 4/6 | 13 | 0 | 0 | 0 |
- R-values are minimums. U-factors and SHGC are maximums.
- The fenestration U-factor column excludes skylights. The SHGC column applies to all glazed fenestration. Remember that the SHGC is the fraction of heat energy passing through the window.
- In Table R402.1.1 above, “3/4” means R-3 continuous insulation on the interior or exterior of the home or R-4 cavity insulation at the interior of the basement
1.4.4.2 R-value computation.
Insulation material used in layers, such as framing cavity insulation and insulating sheathing, shall be summed to compute the component R-value. The manufacturer’s settled R-value shall be used for blown insulation.
1.4.4.3 Access hatches and doors.
Access doors from conditioned spaces to unconditioned spaces (e.g., attics and crawl spaces) shall be weather-stripped and insulated to a level equivalent to the insulation on the surrounding surfaces. Access shall be provided to all equipment that prevents damaging or compressing the insulation. A wood framed or equivalent baffle or retainer is required to be provided when loose fill insulation is installed, the purpose of which is to prevent the loose fill insulation from spilling into the living space when the attic access is opened, and to provide a permanent means of maintaining the installed R-value of the loose fill insulation.
1.4.4.4 Floors.
Floor insulation shall be installed to maintain permanent contact with the underside of the subfloor decking.
1.4.4.5 Basement walls.
Walls associated with conditioned basements shall be insulated from the top of the basement wall down to 10 feet below grade or to the basement floor, whichever is less.
1.4.4.6 Slab-on-grade floors.
Slab-on-grade floors are not required to be insulated in Florida’s Climate Zones, as indicated in Table 402.1.1.
1.4.4.7 Crawl space walls.
As an alternative to insulating floors over crawl spaces, crawl space walls shall be permitted to be insulated when the crawl space is not vented to the outside. Crawl space wall insulation shall be permanently fastened to the wall and extend downward from the floor to the finished grade level and then vertically and/or horizontally for at least an additional 24 inches (610 mm).
Exposed earth in unvented crawl space foundations shall be covered with a continuous Class I vapor retarder in accordance with the Florida Building Code, Building or Florida Building Code, Residential, as applicable.
1.4.4.8 Sunroom insulation.
All sunrooms enclosing conditioned spaces shall meet the insulation requirements of the Code.
1.4.4.9 Air leakage.
The building thermal envelope shall be constructed to limit air leakage in accordance the Code.
1.4.5 Building thermal envelope.
The building thermal envelope shall comply with Sections R402.4.1.1. The sealing methods between dissimilar materials shall allow for differential expansion and contraction.
1.4.5.1 Installation.
The components of the building thermal envelope as listed in Table R402.4.1.1 shall be installed in accordance with the manufacturer’s instructions and the criteria listed in Table R402.4.1.1
Where required by the code official, an approved third party shall inspect all components and verify compliance.
TABLE R402.4.1.1 AIR BARRIER AND INSULATION INSTALLATION
COMPONENT | CRITERIA a |
Air barrier and thermal barrier | A continuous air barrier shall be installed in the building envelope. Exterior thermal envelope contains a continuous air barrier. Breaks or joints in the air barrier shall be sealed. Air-permeable insulation shall not be used as a sealing material. |
Ceiling/attic | The air barrier in any dropped ceiling/soffit shall be aligned with the insulation and any gaps in the air barrier sealed. Access openings, drop down stair or knee wall doors to unconditioned attic spaces shall be sealed. |
Walls | Corners and headers shall be insulated and the junction of the foundation and sill plate shall be sealed. The junction of the top plate and top of exterior walls shall be sealed. Exterior thermal envelope insulation for framed walls shall be installed in substantial contact and continuous alignment with the air barrier. Knee walls shall be sealed. |
Windows, skylights and doors | The space between window/door jambs and framing and skylights and framing shall be sealed. |
Rim joists | Rim joists shall be insulated and include the air barrier. |
Floors (including above-garage and cantilevered floors) | Insulation shall be installed to maintain permanent contact with underside of subfloor decking. The air barrier shall be installed at any exposed edge of insulation. |
Crawl space walls | Where provided in lieu of floor insulation, insulation shall be permanently attached to the crawlspace walls. Exposed earth in unvented crawl spaces shall be covered with a Class I vapor retarder with overlapping joints taped. |
Shafts, penetrations | Duct shafts, utility penetrations, and flue shafts opening to exterior or unconditioned space shall be sealed. |
Narrow cavities | Batts in narrow cavities shall be cut to fit, or narrow cavities shall be filled by insulation that on installation readily conforms to the available cavity space. |
Garage separation | Air sealing shall be provided between the garage and conditioned spaces. |
Recessed lighting | Recessed light fixtures installed in the building thermal envelope shall be air tight, IC rated, and sealed to the drywall. |
Plumbing and wiring | Batt insulation shall be cut neatly to fit around wiring and plumbing in exterior walls, or insulation that on installation readily conforms to available space shall extend behind piping and wiring. |
Shower/tub on exterior wall | Exterior walls adjacent to showers and tubs shall be insulated and the air barrier installed separating them from the showers and tubs. |
Electrical/phone box on exterior walls | The air barrier shall be installed behind electrical or communication boxes or air sealed boxes shall be installed. |
HVAC register boots | HVAC register boots that penetrate building thermal envelope shall be sealed to the subfloor or drywall. |
Fireplace | An air barrier shall be installed on fireplace walls. Fireplaces shall have gasketed doors. |
1.4.5.2 Air Leakage Testing.
The building or dwelling unit shall be tested and verified as having an air leakage rate of not exceeding 5 air changes per hour in Climate Zones 1 and 2. An air change per hour is the movement of an air quantity equal to the interior home volume Testing shall be conducted with a blower door at a pressure of 0.2 inches w.g. (50 Pascals). Where required by the code official, testing shall be conducted by an approved third party. A written report of the results of the test shall be signed by the party conducting the test and provided to the code official. Testing shall be performed at any time after creation of all penetrations of the building thermal envelope.
During testing:
- Exterior windows and doors, fireplace and stove doors shall be closed, but not sealed, beyond the intended weather stripping or other infiltration control measures;
- Dampers including exhaust, intake, makeup air, backdraft and flue dampers shall be closed, but not sealed beyond intended infiltration control measures;
- Interior doors, if installed at the time of the test, shall be open;
- Exterior doors for continuous ventilation systems and heat recovery ventilators shall be closed and sealed;
- Heating and cooling systems, if installed at the time of the test, shall be turned off; and
- Supply and return registers, if installed at the time of the test, shall be fully
1.4.5.3 Fireplaces.
New wood-burning fireplaces shall have tight-fitting flue dampers and outdoor combustion air.
1.4.5.4 Fenestration air leakage.
Windows, skylights and sliding glass doors shall have an air infiltration rate of no more than 0.3 cfm per square foot, and swinging doors no more than 0.5 cfm per square foot when tested by an accredited, independent laboratory and listed and labeled by the manufacturer.
Exception: Site-built windows, skylights and doors.
1.4.5.5 Recessed lighting
Recessed luminaires installed in the building thermal envelope shall be sealed to limit air leakage between conditioned and unconditioned spaces. All recessed luminaires shall be IC- rated and labeled as having an air leakage rate not more than 2.0 cfm when tested at a 1.57 psf pressure differential. All recessed luminaires shall be sealed with a gasket or caulk between the housing and the interior wall or ceiling covering.
1.4.6 Controls
1.4.6.1 Thermostat provision
At least one thermostat shall be provided for each separate heating and cooling system.
1.4.6.2 Programmable thermostat
Where the primary heating system is a forced-air furnace, at least one thermostat per dwelling unit shall be capable of controlling the heating and cooling system on a daily schedule to maintain different temperature set points at different times of the day. This thermostat shall include the capability to set back or temporarily operate the system to maintain zone temperatures down to 55°F or up to 85°F. The thermostat shall initially be programmed with a heating temperature set point no higher than 70°F and a cooling temperature set point no lower than 78°F.
1.4.6.3 Heat pump supplementary heat
Heat pumps having supplementary electric-resistance heat shall have controls that, except during defrost, prevent supplemental heat operation when the heat pump compressor can meet the heating load.
1.4.7 Ducts and Air Handlers
The requirements for ducts and air handlers are described in the following sections.
1.4.7.1 Insulation
Supply ducts in attics shall be insulated to a minimum of R-8. All other ducts shall be insulated to a minimum of R-6.
Exception: Ducts or portions thereof located completely inside the building thermal envelope.
1.4.7.2 Sealing
All ducts, air handlers, and filter boxes and building cavities that form the primary air containment passageways for air distribution systems shall be considered ducts or plenum chambers, shall be constructed and sealed in accordance with the Code and shall be shown to meet duct tightness criteria. Duct tightness shall be verified by testing by a certified energy rater certified to be “substantially leak free” by either of the following:
- Post-construction test: Total leakage shall be less than or equal to 4 cfm per 100 square feet of conditioned floor area when tested at a pressure differential of 0.1 inches w.g. across the entire system, including the manufacturer’s air handler enclosure. All register boots shall be taped or otherwise sealed during the
- Rough-in test: Total leakage shall be less than or equal to 4 cfm per 100 square feet of conditioned floor area when tested at a pressure differential of 0.1 inches w.g. across the system, including the manufacturer’s air handler enclosure. All registers shall be taped or otherwise sealed during the test. If the air handler is not installed at the time of the test, total leakage shall be less than or equal to 3 cfm per 100 square feet of conditioned floor area.
Exceptions: The total leakage test is not required for ducts and air handlers located entirely within the building thermal envelope.
1.4.7.3 Sealed air handler.
Air handlers shall have a manufacturer’s designation for an air leakage of no more than 2 percent of the design air flow rate.
1.4.7.4 Building cavities
Building framing cavities shall not be used as ducts or plenums.
1.4.8 Air-handling units.
Air-handling units shall be allowed in attics for compliance only if the following conditions are met:
- The service panel of the equipment is located within 6 feet (1829 mm) of an attic
- A device is installed to alert the owner or shut the unit down when the condensation drain is not working
- The attic access opening is of sufficient size to replace the air
- A notice is posted on the electric service panel indicating to the homeowner that the air handler is located in the attic. Said notice shall be in all capitals, in 16 point type, with the title and first paragraph in bold:
NOTICE TO HOMEOWNER
A PART OF YOUR AIR-CONDITIONING SYSTEM, THE AIR HANDLER, IS LOCATED IN THE ATTIC. FOR PROPER, EFFICIENT, AND ECONOMIC OPERATION OF THE AIR-CONDITIONING SYSTEM, YOU MUST ENSURE THAT REGULAR MAINTENANCE IS PERFORMED. YOUR AIR- CONDITIONING SYSTEM IS EQUIPPED WITH ONE OR BOTH OF THE FOLLOWING: (1) A DEVICE THAT WILL ALERT YOU WHEN THE CONDENSATION DRAIN IS NOT WORKING PROPERLY OR (2) A DEVICE THAT WILL SHUT THE SYSTEM DOWN WHEN THE CONDENSATION DRAIN IS NOT WORKING. TO LIMIT POTENTIAL DAMAGE TO YOUR HOME, AND TO AVOID DISRUPTION OF SERVICE, IT IS RECOMMENDED THAT YOU ENSURE PROPER WORKING ORDER OF THESE DEVICES BEFORE EACH SEASON OF PEAK OPERATION.
1.4.9 Mechanical system piping insulation.
Mechanical system piping capable of carrying fluids above 105°F or below 55°F shall be insulated to a minimum of R-3. Piping insulation exposed to weather shall be protected from damage, including that caused by sunlight, moisture, equipment maintenance, and wind, and shall provide shielding from solar radiation that can cause degradation of the material. Adhesive tape shall not be permitted.
1.4.10 Service hot water systems.
Energy conservation measures for service hot water systems shall be in accordance with the following sections.
1.4.10.1 Circulating hot water systems
Circulating hot water systems shall be provided with an automatic or readily accessible manual switch that can turn off the hot-water circulating pump when the system is not in use.
1.4.10.2 Hot water pipe insulation
Insulation for hot water pipe with a minimum thermal resistance (R-value) of R-3 shall be applied to the following:
- Piping larger than 3/4 inch nominal diameter.
- Piping serving more than one dwelling
- Piping from the water heater to kitchen
- Piping located outside the conditioned
- Piping from the water heater to a distribution
- Piping located under a floor
- Buried
- Supply and return piping in recirculation systems other than demand recirculation
1.4.10.3 Heat traps
Storage water heaters not equipped with integral heat traps and having vertical pipe risers shall have heat traps installed on both the inlets and outlets. External heat traps shall consist of either a commercially available heat trap or a downward and upward bend of at least 31/2 inches in the hot water distribution line and cold water line located as close as possible to the storage tank.
1.4.10.4 Storage water heater temperature controls.
a. Automatic controls.
Service water heating systems shall be equipped with automatic temperature controls capable of adjustment from the lowest to the highest acceptable temperature settings for the intended use. The minimum temperature setting range shall be from 100°F to 140°F.
b. Shut down.
A separate switch or a clearly marked circuit breaker shall be provided to permit the power supplied to electric service systems to be turned off. A separate valve shall be provided to permit the energy supplied to the main burner(s) of combustion types of service water heating systems to be turned off.
1.4.10.5 Water heating equipment.
Water heating equipment installed in residential units shall meet the minimum efficiencies of the Florida Building Code, Energy Conservation, Commercial Provisions, for the type of equipment installed. Equipment used to provide heating functions as part of a combination system shall satisfy all stated requirements for the appropriate water heating category.
1.4.10.6 Solar water heating systems.
Solar systems for domestic hot water production are rated by the annual solar energy factor of the system. The solar energy factor of a system shall be determined from the Florida Solar Energy Center Directory of Certified Solar Systems. Collectors in installed solar water heating systems should meet the following criteria:
- Be installed with a tilt angle between 10 degrees and 40 degrees of the horizontal; and
- Be installed at an orientation within 45 degrees of true
1.4.11 Mechanical ventilation
The building shall be provided with ventilation that meets the requirements of the Florida Building Code, Residential or Florida Building Code, Mechanical, as applicable, or with other approved means of ventilation. Outdoor air intakes and exhausts shall have automatic or gravity dampers that close when the ventilation system is not operating.
1.4.11.1 Whole-house mechanical ventilation system fan efficacy.
Mechanical ventilation system fans shall meet the efficacy requirements of Table R403.5.1.
Fan Location | Air Flow Rate Minimum (CFM) | Minimum Efficacy (CFM/WATT) | Air Flow Rate Maximum (CFM) |
Range hoods | Any | 2.8 | Any |
In-Line fan | Any | 2.8 | Any |
Bathroom, utility room | 10 | 1.4 | < 90 |
Bathroom, utility room | 90 | 2.8 | Any |
Exception: Where mechanical ventilation fans are integral to tested and listed HVAC equipment, they shall be powered by an electronically commutated motor.
1.4.11.2 Ventilation air.
Residential buildings designed to be operated at a positive indoor pressure (the air pressure inside the home is greater than the exterior air pressure) or for mechanical ventilation shall meet the following criteria:
- The design air change per hour minimums for residential buildings in ASHRAE 62, Ventilation for Acceptable Indoor Air Quality, shall be the maximum rates allowed for residential applications.
- No ventilation or air-conditioning system make-up air shall be provided to conditioned space from attics, crawlspaces, attached enclosed garages or outdoor spaces adjacent to swimming pools or
- If ventilation air is drawn from enclosed space(s), then the walls of the space(s) from which air is drawn shall be insulated to a minimum of R-11 and the ceiling shall be insulated to a minimum of R-19, space permitting, or R-10
1.4.12 ELECTRICAL POWER AND LIGHTING SYSTEMS
1.4.12.1 Lighting equipment
A minimum of 75 percent of the lamps in permanently installed lighting fixtures shall be high- efficacy lamps or a minimum of 75 percent of the permanently installed lighting fixtures shall contain only high efficacy lamps.
Exception: Low-voltage lighting shall not be required to utilize high-efficiency lamps
1.4.12.2 Lighting equipment
- Fuel gas lighting systems shall not have continuously burning pilot
- Hydronic systems shall mean those systems that distribute heating and cooling energy directly to individual spaces using liquids pumped through closed-loop piping and that do not depend on ducted, forced airflow to maintain space
- Entire system in conditioned space shall mean that no component of the distribution system, including the air-handler unit, is located outside of the conditioned
- Ductless systems shall be allowed to have forced airflow across a coil but shall not have any ducted airflow external to the manufacturer’s air-handler
1.4.13 Installation criteria for homes claiming the radiant barrier option.
The sheet radiant barrier or interior radiation control coating (IRCC) options may be claimed where the radiant barrier system is to be installed in one of the configurations depicted in Figure R405.7.1, and the following conditions are met:
a.It shall be fabricated over a ceiling insulated to a minimum of R-19 with conventional insulation and shall not be used as a means to achieve partial or whole compliance with a minimum attic insulation level of R-19. Either a sheet type or spray applied IRCC may be
b.If the radiant barrier material has only one surface with high reflectivity or low emissivity it shall be facing downward toward the ceiling
c.The radiant barrier system shall conform to ASTM C 1313, Standard Specification for Sheet Radiant Barriers for Building Construction Applications, or ASTM C 1321, Standard Practice for Installation and Use of Interior Radiation Control Coating Systems (IRCCS) in Building Construction as appropriate for the type of radiant barrier to be installed.
d.The radiant barrier shall be installed so as to cover gable ends without closing off any soffit, gable or roof
Chapter 2.1: Roofing – Advanced Fabricated Building
Materials, Products, and Systems Testing
This module includes an overview of some of the major materials and products that are used in manufactured and modular houses and have not been covered in Manufactured Construction Technology levels 1 and 2 certifications. These include roofing, flooring, cabinets, and painting. In addition, the required testing after the unit completion for water distribution system, drain/waste/vent system, gas system, air duct system, and electrical system are discussed.
2.1 Roofing
Roofing is a general term used to describe type of roof construction and various kinds of roof materials in buildings. The main function of roof is to provide protection from rain or snow. Selecting the most appropriate roof system for each building and knowing the material options are important because roof is one of the most vulnerable parts of a building. Some roof materials are suitable for fire, high-speed wind, extreme heat, and/or cold weather climates. Besides, the diversity in color and pattern of roof material can satisfy aesthetic needs in building design.
Figure 2.1 Roof slope types
Roof shapes differ from region to region due to climates and the materials available for roof structure and covering. Roof shapes vary from almost flat to steeply sloped. They can be a single flat sheet or an arrangement of slopes, gables and hips. Slope is the incline of the roof expressed as a ratio of the vertical rise to the horizontal run. This ratio is always expressed as inches per foot. A roof that rises 3 inches for every 12 inches of run is said to have a “3 in 12” slope.
The slope of a roof is split up into three categories:
- Low slope: Anything under a 4 in 12 slope
- Medium slope: A 4 in12 slope to an 8 in 12 slope
- High Slope: Anything over an 8 in 12 slope
Residential roofs are typically sloped and can be made of shingle, tile, wood, slate and metal. Manufactured HUD homes and modular homes have typically medium slope and commonly use asphalt shingle as roofing material.
The roof framing is usually manufactured trusses either build in an offsite station or build by another manufacturer. The roof deck is attached to the top of trusses and makes the roof frame ready for installation of roofing system. The basic components of roofing system are roof underlayment, roof shingles, and flashing.
Figure 2.2 The basic components of a roofing system
2.1.1 Underlayment
The roof deck should be covered with a layer of roof underlayment. The underlayment is a water-resistant layer under the shingles throughout the roof to protect the roof from moisture. In some areas, it is common to use an additional waterproofing membrane over the underlayment. Underlayment depends on the type of roof shingles. Asphalt felts are typical underlayment for manufactured homes. Asphalt felts consist of heavy felt paper saturated with asphalt or coal tar which are usually available in 15-lb, 30-lb, and 60-lb with 36-inch wide rolls. Special types of underlayment may be required in specific climate conditions. For example, in areas with less than 25F average daily temperature in January, Ice dam protection is recommended. It is common to use 15-lb felt in manufactured homes.
Figure 2.3 Asphalt felt roll installation
2.1.2 Shingles
Roof shingles are available in various types. Most common type of shingles in manufactured homes are asphalt shingles or composition shingles. Other types that may have application in construction industry include slate shingles, concrete and clay tiles, wood shingles, and metal shingles.
Asphalt shingles are very common roofing material available in a wide variety of colors, textures, types, and weights. Shingles are available in two types of organic shingles and fiberglass shingles. Organic shingles are asphalt-saturated paper felt coated with additional asphalt. Asbestos fiber that was commonly used in organic shingles is now prohibited because of cancer risks and environmental disposal hazard of asbestos. Fiberglass shingles have a base of fiberglass mat that are coated with asphalt. Both types of asphalt shingles have a surface covered by mineral granules. Fiberglass-reinforced shingles provide greater fire resistance and have a better quality in general. Higher quality shingles will last longer and will result in saving money in long term. Fiberglass singles typically will last around 20 to 25 years.
Figure 2.4 Types of asphalt shingles
2.1.3 Flashing and ventilation
Flashing is strips of thin sheets that work as additional waterproofing and make watertight seal at the intersections and transitions in the exterior surfaces. Flashings are usually applied at valleys, eaves, rakes, chimneys, and other penetrations. Flashing material should be compatible with the roof and last the same time. Copper, zinc, aluminum, galvanized steel, and vinyl are the most common materials for flashing. All the flashing materials should be coated with a primer as additional protection.
Figure 2.5 Drip edge flashing
Drip edge is a kind of flashing that is installed along the roof edges. Drip edge is fastened with roofing nails using the same material spaced 8 to 10 inches. Roof eave is a vulnerable area for forming ice dams. Flashing is required along the roof eave to avoid water leakage. The drip edge should have 1/4 to 3/8 inch extension over the roof eave.
Roof valleys carry high volume of water and have a possibility of water leakage. Valleys are generally in two types of open valley or closed valley. Open valleys are those in which the roof- covering material stops several inches short of the centerline of the valley. The valley is protected from moisture intrusion by a metal lining. For open valley, first the felt underlayment is installed on the valley and fastened with nails in two sides. The flashing comes over the underlayment and extends on each side. The flashing is nailed from the edge to the roof. Roof shingles should overlap the flashing for at least 6 inches. Closed valleys are those where shingles on one or both sides of the roof extend across the valley onto the adjacent roof slope. Because valley linings for closed valleys are protected by the shingles, the linings may be made of thick underlayment or thinner metal than that used for open valleys. There are two kinds of closed valleys: woven valley and closed cut valley.
Figure 2.6 Open valley and closed valley flashing
In hot climates, the roof system requires attic ventilation to expel solar-heated hot air from the attic to lessen the building cooling load. Roof ventilation inhale the air from soffit vents and exhale it from gable vents or ridge vents. Some types of box vents or turbine vents are also used in some buildings. In some cases, electric turbines or fans are required for proper ventilation.
Figure 2.7 Roof ventilation
2.1.4. Roof installation
Considering safety issues in applying the roof materials is very important. Injuries may happen when cutting roof materials and respiratory hazards of asphalt-based materials.
The first step of roof installation is preparing the roof deck. The roof underlayment and waterproofing membrane (if required) are installed as well as drip edge and the flashing for eaves and valleys. Usually the process starts by installing the drip edge and valley flashing. The flashing is secured to the roof with nails every 6 to 8 inches. The nails head is covered with asphalt. The underlayment and waterproofing membrane are installed to the roof deck with nails. For the places with the risk of heavy snow, protection against ice dam is necessary. Eave flashing is used to prevent water backup behind any ice dam.
The next step is installation of roof shingles. A standard terminology is used in placing the shingles. Exposure is the distance between two courses of roof shingles. Top lap is the height of the shingle minus exposure. Head lap is the distance between top of the shingle in one course to bottom of shingles of the two top courses.
Figure 2.8 Roofing terminology and nail points
Roof shingles may be placed left to right or right to left depending on the preference of the roofer. It can also start from the middle and continue to both ends. The common method is placement of starter row with the tabs of the regular course of shingles. The exposure part of shingle is cut off and applied in the starter course along the roof perimeter. A course of full shingle comes over the starter course. End of the top course should have 6 inches offset from the one below. Fastening the shingles are based on the recommendations provided by the manufacturer. After applying the roof shingles in both sides of the roof, the roof ridge cap is installed. The ridge cap will finish the roof shingles and cover the nails of the last course.
Figure 2.9 Shingle layout
Chapter 2.2: Flooring – Advanced Fabricated Building
In addition to their aesthetic contribution, flooring components have an important role in protecting subfloor sheathing and controlling interior humidity. Flooring components can be affected by the bowing and sagging movements of the inner structure, moisture, and traffic. Due to these factors, flooring materials should be properly selected and installed. The function of space, repair flexibility, ease of maintenance, and service life are important factors in selecting flooring materials. Four types of flooring materials are common in manufactured homes, carpet, vinyl composition tile (VCT), wood, and ceramic tile.
Figure 2.10 Different flooring materials
2.2.1 Carpet
Carpet is made of thick woven fabric and is one of the most popular materials for floor covering. Some of the benefits of using carpet are warmth and comfort, insulation, noise control, and low price. They can be cut and woven in several different ways, and from a variety of fibers. The main carpet fabric construction methods include tufting, weaving, knitting, needlepunching, and bonding. More than 90 percent of carpets are tufted that consists of the face yarn, primary backing fabric, a bonding compound, and (usually) a secondary backing fabric. The face yarn can be cut pile, loop pile, or a combination of both. The bonding compound is SB latex, polyurethane, PVC, or fabric.
Figure 2.11 Cut pile and loop pile
Carpets are classified based on their different properties, such as pile yarn weight, pile thicknesses, backing, surface texture, and number of tuffs per square feet. Pile yarns can be made with many materials, such as nylon, olefin (polypropylene), acrylic, polyester, wool, or cotton. Wool has been used for many years and is proved to have good performance. However, synthetic fibers are receiving more attention recently since they have a variety of high quality and durable products with an affordable price. The production process for synthetic fiber includes extruding a jet of molten polymer (or polymer solution) through tiny hole of a spinneret. The filaments are cooled down in different shapes and with different specifications. These fibers are used in carpet production based on their required properties.
Figure 2.12 Carpets have wide variety of colors
The Department of Housing and Urban Development (HUD), through the “Use of Materials Bulletin Um-44d”, does not allow the use of carpet in baths, kitchens and service areas, such as laundry, utility and furnace rooms.
Carpet installation needs proper es ma ng and coordination. Considering the architecture drawings of the building, carpet loc on and direction is recognized. Before the installation for around 48 hours the room temperature should be (65 ̊ to 95 F) and the surface temperature should be around 65oF. The humidity should be around 65% and ventilation is required during and 48 to 72 hours after installation.
Carpet can be installed over finished flooring, concrete, plywood, particleboard or any other smooth surface. The sub-surface should be clean, dry, free of contaminants, high quality and leveled properly. Vacuuming is required to remove dust from the floor area.
The two main methods of installation are stretch-in and glue-down. The stretch-in method is more common in residential buildings. In this method, a power stretcher is used to stretch the carpet and put it in place. Tack strip is mounted around the perimeter. Tack strips are pieces of plywood with rows of metal pins designed to grip carpet. There are variety of tack strips with specific uses. Padding is placed between the tack strips with appropriate adhesive. Carpet is placed above padding and stretched until it is attached to strips. Adhesive or heated seaming iron shall be used for seaming. Seam placement is critical for successful installation. Seams may be held in place after edge securing by hot-melt tape, tape and latex, hand sewing, or other methods.
Figure 2.13 stretch-in installation
Figure 2.14 Seam placement
The glue down method includes direct glue-down and double glue-down. In direct glue-down, the carpet is directly attached to the floor with an adhesive. The carpet may have attached cushion. In double glue down method, first the cushion is attached to the floor, and then the carpet is bonded to the cushion with adhesive material.
2.2.2 Vinyl flooring
Vinyl flooring is the most popular type of flooring for manufactured construction. It is made with ingredients that are mixed under high temperature and pressure. All vinyl flooring is composed of polyvinyl chloride (PVC) resin along with additives, such as plasticizers, stabilizers, pigments, and fillers. Manufactured processes provide a smooth and decorative flooring, with different thicknesses and dimensions. Vinyl components are available in vinyl sheets or vinyl composition tiles (VCT).
Vinyl flooring is highly resilient, durable and resistant. However, it can be affected by several job conditions, preventing vinyl to properly bond with the subfloor structure. Subfloor sheathings should always be firmly fastened, smooth, and free from dust and moisture. Also, it is recommended to maintain surface temperature between 70oF and 90oF for around 48 hours before, during, and for 48 hours after completion of VCT installation. The room should have good ventilation during installation.
VCT is composed of binder, fillers, and pigments. The binder is made from poly (vinyl chloride), or vinyl chloride copolymers, or both, compounded with suitable plasticizers and stabilizers. ASTM F1066 covers VCT for flooring application. They are classified into three classes including Class 1 for solid tile, Class 2 for through pattern tile, and Class 3 for surface pattern tile. Solid color tile is uniform. In through pattern tile, the color or pattern is the same throughout the tile. In surface pattern tile, the pattern remains only on the surface. Tile products range in size from 9 by 9 inches up to 36 by 36 inches, and in several gauges, colors and patterns. Usually, vinyl tiles have self-adhesive on the back, which facilitate installation. The VCT is available in market based on class, color, pattern, and wearing surface.
The design layout for VCT installation shall be decided by laying down some tiles cross over the room which could be either in square or diagonal configuration. Adjustments shall be made if the layout results in tiles with less than half original dimension. A chalk line from center of each side of the room is required to determine the center point of the area. The intersection of the chalk lines shall be square at 90°. Placement begins by applying recommended tile adhesive with notched trowel at the intersection of chalk lines. The adhesive needs a sufficient open time before laying the tile. Tiles shall be placed with tight, straight and aligned joints in both directions. The placement may be based on square or diagonal Layout. Borders and fixed objects may require scribing for fitting. Heating the tile with a heat gun or blowtorch may facilitate the cutting process.
Figure 2.15 VCT placement with square and diagonal Layout
Vinyl Sheets installation needs a plan in the area to minimize the number and length of seams. Sheets seams shall be perpendicular to the subfloor joints. The vinyl sheet is applied in the room area by adding at least 3 inch each side before cutting and placing. In the seam area, the top sheet should overlap the previous sheet by approximately 1/2 inch. The excess of vinyl near of walls is cut and the sheet is fixed by scribing the surface format. Special adhesives shall be used for room perimeter, seam lines and columns. The recommended seam adhesive is applied after placement in a 3 to 6-inch-wide band, centered under the seam.
2.2.3 Wood
Wood flooring is a natural and classy style flooring that can satisfy aesthetic desires in the home decoration and be compatible with traditional or modern home style. Wood floors are very durable. They are available in many species with various color, grain, and physical characteristics. There are more than 50 domestic and imported species of wood flooring available. The cut angle of the wood determines how the finished product looks. The four common cut angle include plainsawn, quartersawn, riftsawn or livesawn. Plainsawn is the most common cut. Other cuts are more expensive and provide different grain looks.
Figure 2.16 Different cut for wood products
Wood or components must comply with specific grading rules for a particular wood type. For example, the National Oak Flooring Manufacturers Association (NOFMA) regulates the production of oak flooring through the Official Flooring Grading Rules, while the Maple Flooring Manufacturers Association (MFMA) regulates for maple, beech and birch floorings. Wood flooring are made in three basic styles: strip, plank and parquet. They are made from solid or engineered boards. Strip flooring is less than 3” wide. Plank flooring is equal to or greater than 3” wide. Both strip and plank flooring are linear but strip flooring provides wider view and make the room look larger. Parquet flooring is available in various sizes, and provides a geometric, non-linear look.
Normally, hardwood flooring are the last components of manufacturing construction, mostly installed only in the owner’s site. The subfloor must be flat, clean, dry before installation. Average moisture should be within the range as specified by the product manufacturer. In some cases, vapor retarder is required on the subfloor before applying the adhesive. The wood floor is not recommended for installation below grade.
Strip flooring are installed in parallel rows which have a standard pattern with side-matched and end-matched methods (tongue-and-grooved). They are commonly installed with blind nail- down directly to wood or plywood subfloors, or over wood screeds on concrete slab construction. The first row need both top nail and blind nail. Some types are glued directly to the subfloor with paste-like adhesives.
Planks flooring is installed in parallel rows. It is available in end-matched and side-matched with same thicknesses as strip floorings. The installation should follow manufacturers recommended procedure. It is important to avoid “H” pattern and end joint should be staggered row to row a minimum of 6” for strip flooring, 8-10” for 3” to 5” plank, and 10” for plank wider than 5”.
Figure 2.17 Avoid “H” joints and stagger end joint
Parquet flooring is divided into single slats or blocks. Single slats are individual strips of wood available in side- and end-matched strips, with lengths ranging from 6 to 18 inches, and in all types of woods, grades and sizes as strip flooring. Blocks can be unit, laminated or slat. Unit blocks are short strips joined together to form a square unit. Laminated blocks are made by many plies of veneer bounded with adhesive. Slat blocks or mosaic parquets use narrow slats of wood preassembled into larger units.
Figure 2.18 Different styles of flooring
Parquet lay out can be in several ways including square layout from center of the room, square layout from the wall, using wall layout, diagonal layout, and herringbone layout. Two chalk lines are drawn with square 90° angle and the parquet tile is placed at the intersection of the two chalk lines. Proper placement of the first floor tile is the key to the entire installation.
For diagonal layout, a diagonal chalk line is used with 45° angle from the chalk line intersection. For drawing the diagonal line, 4-feet distance is pointed on the main line from each side of the intersection. The points are connected to shape a 4-feet length square. The diagonal line is resulted by continuing the diameter of this square from both directions. Parquet tile is placed throughout the chalk line. For herringbone layout, one center line and two working lines are required. For herringbone installation, a square piece of plywood with the same size as parquet tile is prepared. The piece is placed at the starting point of the bottom chalk line and fixed.
Parquet tiles are then properly placed in the location and fastened to the subfloor.
Figure 2.19 Square and diagonal layout
Figure 2.20 Herringbone layout and installation
2.2.4 Ceramic tile
Ceramic components are made with natural clay and non-metallic minerals heated at high temperature and manufactured in modular units and sizes. The clay is pressed into a shape, glazed and then fired in a kiln. Products varying in dimensions, proprieties, and appearance. The glazing is from glass and metal elements which make the color and creates a hard, shiny surface. There are glazed wall tiles, mosaic tiles, paver tiles, quarry tiles and decorative tiles.
The surface may have texture or raised design to make slip-resistant. Generally, ceramic tiles have high abrasion and moisture resistance, making them a good choice for interior finishes. Tiles are applied on a tile backer and on top of subfloor. A thin mortar layer is applied on the subfloor as a setting for tile backer and on top of tile backer as a layer for setting tile. Tile backer is a sheet panel that is installed on subfloor as underlayment for the installation of tiles. Cement board is a common tile backer. In some cases, especially for wet area and steam room, tile underlayment membranes are required to provide additional sound abatement, waterproofing, or moisture control.
Figure 2.21 Different layers in a typical ceramic tile floor
Setting product shall be selected according to the environmental condition. Cement mortar, dry-set mortar, Latex-Portland cement mortar, epoxy mortar and organic adhesives are the most used types of setting products. Mortar contains dry-polymer additives and should be mixed with water. Latex-modified mortar is produced by mixing dry-set mortar with a liquid latex additive.
American National Standards Institute (ANSI) publishes specifications for the installation of ceramic tile and American Society for Testing and Materials (ASTM) publishes standard test methods for tile setting materials.
Substrate floor must be leveled, clean, and free form dust before installation. In case of using a back layer, it should be fastened into the subfloor properly, according to the manufacturer’s recommendations. A layout plan similar to vinyl tiles is recommended. Tile cuts should not be smaller than ½ tile. Tiles are placed in the area covered with mortar. Grouting should be settled after ceramic installation. The floor grout is prepared and poured over the tile after adhesive is dry. The grout is forced into the joints with a rubber float trowel. A grout float is used to clean tiles surface and remove the excess grout.
Chapter 2.3: Cabinets – Advanced Fabricated Building
Kitchen cabinets are the built-in furniture installed in many kitchens for storage of food, cooking equipment, silverware, and dishes. They are available in three grades, including stock, semicustom, and custom units. Stock or modular cabinets are mass produced in factory. They are available as disassembled or preassembled units and are installed by a carpenter. Using preassembled units is more common in manufactured homes. Semicustom cabinets are more expensive products, produced in a cabinet shop and available in several styles and in a variety of standard sizes. Custom cabinets are made-to-order units to satisfy a specific application.
2.3.1. Cabinet components
A basic kitchen cabinet has three main units including base cabinet, wall cabinet and countertop. The base cabinet is composed of some components attached together and fastened to the wall. It is typically 34 1/2” high and 24″ deep. The countertop is a top surface for base cabinet with typical thickness of 1 1/2” and depth of 25″. Wall cabinet are attached to the wall on the top of countertop with a distance between 15″ to 18″. Based on building codes, the wall cabinet should be placed at least 24″ to 30″ above the cooking unit and 22″ above the sink. Wall cabinets are available with typical depth of 12″ and typical height of 30″. However, shorter cabinets are available for specific proposes such as above sinks, refrigerators, and stoves.
Most cabinet systems are attached to wall. However, there are some freestanding cabinets called island. The islands should have enough space to allow door swing or access to large appliances. The ideal distance between the islands and other cabinets is 36″ to 42″. In some cases, islands may need a supporting frame.
Cabinet units consist of doors, drawers, and adjustable shelfs. They may need framework for additional support. Frameless cabinets are called European or Euro-style cabinets. Hinges, knobs and pulls, and door catches are some other components used in the cabinets which are available in a variety of types, shapes, sizes, and finishes. Countertops covered with plastic laminate or with natural or synthetic solid-surface materials are two common countertops from several available types. Countertops covered with plastic laminate are durable, affordable, easy-to-clean, and available in various colors and patterns. Solid-surface countertop is becoming popular in professionally designed kitchens and baths due to its attractive appearance and durability.
Figure 2.22 Components of typical kitchen cabinets
Figure 2.23 Hinges, knobs and pulls, and door catches
2.3.2 Cabinet Materials
Cabinets are made from various materials with various quality and price. Cabinets made from panels of particleboard with a vinyl film or melamine applied to exposed surfaces have low quality and low price. Cabinets made from veneers and solid hardwoods such as oak, cherry, birch, ash, or hickory have high quality. Interior panels and drawer bottoms are typically made from hardboard, particleboard, plywood, or medium-density fiberboard (MDF). Visible exterior layer such as doors, drawers, and frames are made from variety of softwoods and hardwoods. Some of the woods commonly used in cabinet construction are ash, beech, birch, cherry, oak, maple, and pine.
The plastic laminate countertop has a core material, generally medium-density fiberboard (MDF), plywood, or particleboard covered with a layer of plastic laminate. Plastic laminate is made of layers of resin-impregnated craft paper with a top layer of colored melamine.
Figure 2.24 Typical countertop with plastic laminate cover
2.3.3. Cabinet Installation
Before the cabinet installation, the underlying surface must be properly prepared. A backing boards should be installed in the proper location to support wall cabinets. The installation starts from the corner units of wall cabinets. For making the cabinet layout on the wall, the position of all wall studs should be marked in the wall area with a plumb line. A long straightedge 4’ level is used to find the highest spot in the floor. A chalk line is drawn from the spot for where the bottom of base cabinet should be installed. Other chalk lines are drawn for top of base cabinet, and bottom and top of wall cabinet.
Figure 2.25 Reference lines for Cabinet layout
After marking the layout for stud location, the points at top and bottom cabinet mounting rails should be predrilled. It is required to countersink the drilled holes on the mounting rails.
Cabinet placement should be according to the chalk line. A T-brace or a temporary cleat is required as temporary support for wall cabinets.
Figure 2.26 T-brace or temporary cleat as temporary support for wall cabinets
Next step is installing the base cabinets. The reason to start from wall cabinets is to provide better access and prevent injuries during installation. The installation of base cabinets is similar to wall cabinets. However, shim is used in the installation to make the horizontal frame members level and straight. Finally, the countertop is placed on top of the base cabinets and fastened to the wall.
Figure 2.27 Shimming base cabinets for leveling
Chapter 2.4: Painting – Advanced Fabricated Building
The terms paint and coating are used interchangeably and there is no accepted definition that explain their difference. In industry paints are air-drying materials applied as liquid in the field. The paint system contains several products such as primers, emulsions, enamels, opaque stains, sealers, fillers, and other materials used as prime, intermediate or finish coats. Unlike paints, many coatings do not contain pigments and may not harden completely. Special coatings are thick and with high performance.
The main purposes of applying paints to a surface are protection, decoration, or to serve a particular function such as weatherproofing, etc. Paints are liquid materials that could be applied by brush, roller, or spray. Paints are composed of pigments, solvent, and binders. Most paints are described according to the solvent used, which could be alkyd, latex, oil, oleoresinous, rubber, or urethane. For a professional paint job, it is required to select the correct paint, prepare the surface, and apply the paint accurately.
2.4.1. Components
All coatings and paints are composed of the four basic ingredients including solvents, resins, pigments, and additives. The paints are also known as a combination of pigments and a vehicle. The vehicle consists of the resin or binder and a suitable solvent or water. The paint will be ready after adding and mixing the pigment particles and any additives into the vehicle. Various paints and coatings categories such as paints, stains, clear coatings, and special coatings are produced with different combination of these four basic ingredients.
The paints are grouped in two types of water-based and oil-based paints. In the water-based paints, also known as latex paints, the vehicle is an emulsion of water and suitable resins. In the oil-based paints, or alkyd paints, a natural or synthetic (alkyd) oil resins is dissolved or dispersed in a solvent. Some water-based paints have small amount of solvent in the vehicle. Water- based paints are easy to apply, have little odor, are easily removed with water, dry quickly, have good resistance against cracks, and do not lose color over time. However, oil-based paints have an attractive gloss, create a smooth finish, tolerate poor surface preparation, perform well at lower temperatures, and have a more durable finish.
Nowadays, there is a strong shift away from oil-based paints in the industry because of the potential environmental and health problems caused by toxic substances in oil-paints. The reason is to eliminate or reduce the use of volatile organic compounds (VOCs) and lead. Most paint solvents are VOCs which evaporate after paint application. VOCs evaporation forms a chemical reaction with nitrous oxides and sunlight to form ozone and air pollutants. Besides, VOCs have negative impact on indoor air quality of the buildings. As a result, there are many restrictive VOCs legislations, and paint manufacturers are aiming to reduce or eliminate the amount of VOCs solvents in their products.
Figure 2.28 Basic components of coatings
Pigments consist of two or more elements combined to form an organic or inorganic compound. They give the paint its color, level of sheen or gloss, and opacity. Opacity is the ability of paint to cover or hide the underlying surface. Adding more pigment in the coating results in more opacity. Finer ground particles provide better opacity and smoothness.
Inorganic pigments are usually made from simple oxides of metal such as iron, zinc, or chromium. Titanium dioxide is the most common inorganic white pigment. Organic pigments are carbon-based chemical compounds. They are typically derived from coal tar and petroleum. Pigments are available for various functions including color pigments, hiding pigments, extender pigments, barrier pigments, inhibitive pigments, and sacrificial pigments.
Additives are usually added in small quantities to the paints for specific purposes and to improve its performance. Common types of additives are driers, anti-skinning agents, fungicides, thixotropes, flow agents, emulsion aids, UV absorbers, and plasticizers.
Resins or binders are used to keep pigment and additives together and attach them to the substrate. The type of resin determines durability and lots of other properties of the paint. Resins may be natural or synthetic. Natural resins are thermoplastic, so heating will make them soft and deformed. Some common natural resins are copal, lac, rosin, dammon, and manila.
Synthetic resins are produced in manufacturing processes. They have good resistance to moisture, chemicals, and UV light. So, they are more widely used in both water-based and oil- based paints. Acrylic, alkyd, latex, and vinyl resin are some of the common synthetic resins.
Figure 2.29 Common resins used in coating
Solvents are liquids used in oil-based coating to dissolve the pigments and additive particles. They reduce paint viscosity for easier application. After applying the paints to a surface, the solvent is evaporated and film formation process will start. In this process, paint becomes a dry, stable, and solid surface. Solvents are grouped into five types including hydrocarbon solvents, turpentines, ketones, esters, and alcohols. It is common to use a combination of solvents in a paint. The solvent combination is used in a paint to reach desired properties including consistency, leveling, drying, adhesion, and durability.
The film formation process by which a paint dries and cures is determined mainly by the characteristics of its resins. Film forming may happen in three processes including oxidation, evaporation, or chemical crosslinking. Oxidation is more common for oil-based paints in which after evaporation of the solvent, surface of resin will form a chemical reaction with the oxygen in the air and make a dry and stable surface. In case of water-based paints, water acts to disperse the pigments and additive particles to make an emulsion. The evaporation is more common for water-based paints in which the coating is converted to a solid film after water evaporation. No chemical reaction is involved in this process. In chemical crosslinking a chemical reaction happens to provide the final surface.
Figure 2.30 Film forming with evaporation and oxidation process
2.4.2 Classifications
There are different ways to classify paints and coatings. The most common system for architectural and industrial paints is classification based on the vehicles or binders used in the paints. There are four categories in this system including alkyd, latex, epoxy, and urethane.
Alkyd paints are driven from combination of an oil acid with alcohol. Latex paints are emulsion of acrylic or vinyl acrylic synthetic resins and pigments kept in water. Epoxy paints and urethane paints are driven from an epoxy resin and urethane resins, respectively.
Manufacturers have another common system for classification of coatings in which the function and end use of coating is considered. These classifications are:
- Primers/undercoats
- Tie coats
- Finish coats
- Sealers
- Shellacs, varnishes, and lacquers
- Stains
- Special purpose coatings
Primers are the first layer of coating in a multi-coat system. They provide adhesion and protect the substrate. Tie coats are a transition from a primer or undercoat to a finish coat. A finish coat, or top coat, is the last layer of coating applied in a multi-coat system. They provide the desired appearance as well as protect primed substrate from abrasion, heat, cold, sunlight, moisture, water immersion, or damaging chemicals. In some cases, sealers are applied before the primer in a porous surface. Shellacs, varnishes, and lacquers are usually applied to provide finish look for furniture and interior surfaces. Stains are applied before varnishes to provide better appearance and clear finish. Finally, there are many special purpose coatings made for specific applications. Some of these include fillers, texture coatings, elastomeric coatings, dry, fall/fog coatings, floor coatings, fire-retardant/high heat-resistant coatings, and encapsulants.
2.4.3 Tools
Different kinds of brushes and rollers are used to apply paint. The brushes are usually used for painting small areas, cutting in, or windows and doors. Cutting in is painting inside corners and around the trim edges before applying paint with a roller. Rollers are typically used for painting large, flat surfaces such as walls, ceilings, and floors. Painting is much faster with roller than with a brush.
All paint brushes are composed of bristles, a heel, a ferrule, and a handle. The brush may have a very poor to excellent quality based on its materials and construction. Brushes are made for specific propose and performance. Brushes are composed of natural or synthetic bristles. Hogs’ hair is a common natural bristle which hold more paint and spread it evenly onto a surface.
Generally, natural bristles are used only with oil or alkyd paints and synthetic bristles are used with water-based paints. Some common types of brushes are wall brushes, varnish brushes, sash and trim brushes, stain brushes, decorative brushes, and special purpose brushes. Selecting a brush should be based on the desired type of finish, the size and shape of the area being covered, and the quality and speed of application.
Figure 2.31 Parts of a brush
Rollers are covered with a fabric component to easily transfer and spread paint onto a surface by rolling. A good roller should apply highest amount of paint on the surface without leaving roller marks, craters, bubbles, and other surface imperfections. They are classified into dip rollers, self-feeding rollers, and special purpose rollers. This classification is based on the method of supplying paint to the roller cover. Dip rollers are the most common type of roller. The roller is dipped into the roller tray or a five-gallon can to supply paint to the roller cover. Dip rollers are composed of a handle, a frame, and a cage or cylinder which holds the roller cover. In self-feeding rollers, the roller cover is connected to a continuous supply of paint which eliminates the need for dipping. Self-feeding rollers are the most efficient types for working in a larger scale. There are some other kinds of rollers for special purposes such as trim rollers, pipe rollers, paint stripers, and spike rollers.
Figure 2.32 Parts of a dip roller
Figure 2.33 Types of Self-feeding roller
2.4.4 Application
The painting system and type of coating is selected based on the surface material and area of working. There are different considerations for wood, metal, masonry, or other interior and exterior surfaces. Usually, manufacturers of building materials have recommendation for the desired coating. The process of paint application includes preparing the surface or substrate, protecting adjacent surfaces, preparing the paints, applying the primer, sealing and filling cracks and joints, and applying the topcoat paint.
Preparing the surface includes cleaning, repairing, and pretreating (if necessary). Detergents, solvents, and chemical treatments are some materials used for cleaning surfaces. Different types of surfaces may need specific preparation. For any surface, proper detergent or solvent is used to clean the surface from any contaminants. All surfaces should be dry and vacuumed to remove any dust. It is important to properly seal the spots and stains as well as fill any hole and crack on the surface. The painting surfaces in a building are wood, metal, plaster, drywall, masonry or cementitious, and synthetic.
Wood surfaces should have moisture of 15% or lower. Sunlight may cause some ultraviolet damages in the wood, so the new wood should be primed shortly. Concrete surfaces need at least 28 days to finish curing concrete. ASTM D-4258 is a standard practice for cleaning concrete. Laitance and efflorescence should be removed from the surface. For steel surfaces, it is important to remove slag, flux, weld spatter, and rough edges. Any contaminates including rust, dirt, mill scale, and old paint should be removed from the surface using hand tools, power tools, blast machines, or water jetting machines. For drywall surfaces, joint compound is used to cover nail and screws in the attachments and seams. The joint compound should be dried and sanded before coating. In case of plaster surfaces, if any panel was loose or broken it should be repaired, replaced, or hardened with a white vinegar wash. The surfaces should have moisture of 6% or lower and its alkaline level should be neutral.
Sealants are used to protect the surface from moisture, air, and heat. Fillers are applied to the substrates to fill any holes, cracks, tears, and other spaces. Caulking is a general term to describe any sealant or filler that is available in cartridges. Caulks and putties are actually those sealants and fillers used on construction joints. Sealant may apply on different surfaces such as wood, metal, and, ceramic. They are usually applied after the primer and before topcoat painting.
Preparing the paint is to provide uniform color and consistency. It is done by mixing all the components properly. All surrounding surfaces and areas should be protected to avoid any demand. In painting with a brush, the bristle is immersed in paint container halfway and the paint is applied to the surface. For applying paint with rollers, after loading enough paint to the roller cover, it follows zigzag patterns like the letter N or M on the surface.
Panting interior surfaces should begin from a corner of the ceiling. It will start from the top toward the bottom. In case of multi-coating, the existing layer should be dry before applying the next coat. Cutting in and trimming is done with a brush tool and the surface coating is applied with a roller. Cutting in is painting corners and the perimeter of windows and doors before using roller. The reason for applying cut in layer is to keep the wet edge and prevent
lapping. Keeping wet edge is to start the unpainted area when the adjacent painted surface is still wet. The reason is to avoid lap marks and feather the edges. The roller should be applied on the cutting area to make the uniform texture all over the surface. After ceiling is done, wall painting is started. The coating is from top of the wall to the bottom and usually from left side of the room to the right.
Exterior surfaces follow the same method as the interiors. It is common to start from main wall surfaces. Other painting areas are trim, railings, porches, steps, and the foundation. The environmental condition should be ideal for painting. Temperature should be in an accepted range, suggested by the manufacturers. Sunlight should be enough to dry the surface but not too much to make it dry too fast. Rain or strong winds may cause some damage or stain in the painting surface. Same as interior walls, rollers are used for painting large flat surfaces made from brick, stucco, concrete, or masonry. For overlapping siding such as clapboards or shingles, first a brush is used to cover bottom edges. Next the rollers cover the top surface. The painting starts from a painted wet siding to the adjacent unpainted surface. The surface is coated with smooth, even horizontal strokes. The final brush stroke should be vertically along the grain of the wood.
Chapter 2.5: System Testing – Advanced Fabricated Buildings
To ensure safety, usability, and functionality of the manufactured building components as well as the final unit, a series of tests and inspections are performed in every manufactured home as shown in Table 1. The testing and inspection procedures establish systematic ways to assure product conformance to specified requirements and are helpful to detect problems and avoid quality failures.
The tests and inspections are listed on the traveler in predetermined sequences and are completed in accordance with the company procedure and design manual, which are based on the applicable codes and standards. When no specific applicable procedure is defined, the tests should comply with the HUD standards (for HUD homes) or the local codes (for modular homes). The traveler indicates what needs to be tested/inspected, and will be marked by the inspector to confirm the reliability of that section. Third party inspectors will have access to the traveler for monitoring purposes. The majority of inspections are completed by the internal audit department. The findings are documented and corrective actions are taken to resolve the noted issues. A failure in a system requires repair and re-testing.
Due to the large geographical area served, the travel time involved, and a possible need for corrections, the majority of the tests are conducted in the factory environment. Most tests should follow a specific procedure that might vary in details according to the local codes and regulations. Various tools are needed for each test and several walk-through inspections take place to verify the performance of building systems. The tests shown in Table 1 are explained in the following sections.
Table 1: System tests
Water distribution test |
DWV-flood test/fixture test |
Gas piping test |
Duct system air-tightness test |
Dielectric strength test |
Electrical continuity test |
Electrical operational test |
Electrical polarity test |
2.5.1 Water Distribution Test
Purpose: To ensure the water distribution system, including piping and fittings, is properly installed free of leakage.
Equipment: A pressure gauge
Figure 2.34 Water pressure test
Procedure: After water lines and their connections to fixtures are completed, the system is pressurized with air or water or a combination of both up to 100 psi. The starting pressure is recorded. After 15 minutes the pressure is re-recorded. A significant pressure drop over 15%) indicates a failure in the system. A small pressure drop (less than 15%) may be due to line expansion. In that case, the pressure must be set back to the original 100 psi and re-recorded after 15 minutes. Note that all valves should be kept close and water heater can be filled with water or bypassed by connecting the hot and cold water lines together. Alternatively, the water heater may be unplugged by use of caps on the end of the lines.
2.5.2 Drain, Waste, & Vent (DWV) and Fixture Tests
Purpose: To test the system for leakage and retarded flow
Equipment: Water source, removable plugs, recycling pump tester optional
Figure 2.35 DWV and fixtures Source: Courtesy of Denny Plumbing
Procedure: After all connections and drain lines are installed the waste and vent system have to be tested by one of the three following methods for evidence and indication of leakage.
- Water test. Before plumbing fixtures are connected, all of the opening into the piping have to be plugged and the system subjected to a static water test for 15 minutes by filling it with water to the top of the highest vent opening. There shouldn’t be any evidence of leakage.
- Flood level test. After all fixtures are installed, the drain system is filled with water to the level of the toilet bowl or to the clothes washer standpipe using a hose. The water level in each toilet is noted. Water levels should not be less than 3 inches. After all trapped air has been released, the test is hold for at least 15 minutes. The system is checked for any evidence of leaks. A drop in the water level in toilets or designated fixtures is an indication of system failure.
- Air test. First all DWV systems that are plugged into piping system are filled with water and the entire system is pressurized with air to a 2-inch water column load (0.072 psi). The air pressure is kept for 3 minutes. In case there is a loss of pressure, the leakage location can be identified by pumping smoke into the system, or with the use of soapy water to the exterior of the system.
2.5.3 Gas Piping Test
Purpose: To test the gas piping, risers and valves for leaks
Equipment:
- A gauge, mercury manometer, or similar device
- A source for air to provide continuous flow at pressure.
Figure 2.36 Gas test gauge Procedure:
The gas system is pressurized with air to a minimum of 3 psi or 48 oz per square inch or 14 inches water column or 6 inches of mercury column according to the HUD code. Once the pressure in the system is kept for at least 10 minutes without any drop, the test is complete. The leakage can be checked with soupy water and bubble test. Where leakage is located, the affected portion of the piping system shall be repaired or replaced and retested.
2.5.4 Duct System Air-Tightness Test
Purpose: To verify air-tightness of HVAC duct systems Equipment: A water manometer or equivalent device
Figure 2.37 Duct system air leakage test
Procedure: A supply duct system is tested for the air-tightness by applying static pressure to the duct system. All registers should be sealed and the furnace air circulator should be at high speed. The static pressure in the duct system should be at least 80 percent of the pressure measured in the furnace casing. Pressures are measured with a water manometer or an equivalent device.
2.5.5 Dielectric Test
Purpose: To determine the effectiveness of electrical components insulation. The dielectric test verifies the integrity of all branch circuits.
Equipment: AC or DC Dielectric Tester. The plant may choose to use either an AC or a DC test
Figure 2.38 Dielectric Tester
Preparation: The test is performed after all branch circuits and wiring work are completed. All circuit breakers and switches are kept in the “on” position. In the AC dielectric test, water heater, furnace, and any other similar appliances are kept disconnected. For the DC dielectric test, direct-wired appliances (except electric water heaters), smoke alarms, motion detectors and fluorescent light fixtures may remain connected. In order to ovoid electrocution, it is important to make sure no one is working on, or is in contact with any metal substance that may become energized.
Procedure: The dielectric test is applied by injecting high voltage to the electrical system and monitoring current drop with the use of dielectric tester. The wiring system shall resist current drop when 1080 to 1250 volts-ac (1527 to 1768 volts-dc) is applied for a minimum of one second, not to exceed three seconds; or alternatively, when 900 to 1079 volts-ac (1273 to 1526 volts-dc) is applied for a minimum of 60 seconds, not to exceed two minutes. Fixtures and other appliances are not required to withstand the dielectric strength test. However, the test is performed after they are installed and branch circuits are complete.
2.5.6 Continuity Test
Purpose: To ensure that metallic parts are properly bonded. It checks if an electric circuit is in fact a complete circuit.
Equipment: Continuity Tester
Figure 2.39 Continuity test
Procedure: The test is conducted after all electrical wiring and the bonding of all non-current carrying metal parts are completed. Connect one contact from the tester to an accessible ground, and the other contact to each part being tested. The test indicator should indicate “on” if the part is bonded. Example of non-current carrying metal parts to be checked are:
- The steel chassis.
- Metallic raceways.
- Metal siding.
- Metal roofs.
- Metal HVAC ducts (foil covered insulated ducts do not need to be checked).
- Metal gas piping.
- Appliance enclosures for fans, water heaters, furnaces and fireplaces.
2.5.7 Operational Test
Purpose: To ensure all electrical equipment is properly connected and in working order.
Equipment: GFCI Tripping Device, GFCI Circuit Tester (Note: this test may be accomplished concurrently with the polarity test.)
Figure 2.40 Operational test
Procedure: The purpose of the operational test is to verify that all equipment, except water heaters, electric furnaces, dishwashers, clothes washers/dryers, and portable appliances, are connected and in working order. After all electrical connections and devices are installed, electric power is supplied to all circuits in the home. Except for appliances such as a stove, range, washer, dryer, water heater, furnace, air conditioner, etc., all others as well as any receptacle outlet should be tested. Outlets that are Ground Fault Circuit Interrupter (GFCI) protected are to be tested with a GFCI tripping device. All bathroom receptacles, outside receptacles, all kitchen receptacles serving counter tops, receptacles within 6-feet of a wet bar sink and hydro-massage tubs must be GFCI protected. Direct wire hydro-massage tubs will require GFCI breakers that may be tested by pushing the test button on the GFCI breaker.
2.5.8 Polarity Test
Purpose: To determine that all electrical wiring connections have been properly made.
Equipment: Polarity Checker
Figure 2.41 Polarity test
Procedure: Having completed all electrical connections and installed electrical devices, electric power is supplied to all circuits. Then the polarity checker should be plugged into each 120-volt receptacle outlet, including the outside and heat tape receptacles and observe the indicator lights for the correct polarity. In case a switched receptacle outlet is used, the switch should be in the “off” mode at the beginning of this test so that the function of the switch and receptacle combination can be verified. Turn the switch “on” after making sure the switched receptacle is not energized when the switch is “off”. The electrical connections that cannot be checked with a polarity checker shall be checked by visually inspecting the connections prior to covering.
Chapter 3.1: Panelized Buildings – Advanced Fabricated Building
3.1.1 Definition
Panelization is the process of manufacturing prefabricated exterior and interior wall assemblies under controlled environmental conditions using light wood or light-gauge metal framing. The panels are prepared using cutting-edge automation, assembled according to the design specifications, and then packaged for transportation. Once at the jobsite, panels can be installed by a crew and cranes (Fig. 3.1).
Figure 3.1 Installation of a panelized wooden wall.
The highest efficiency for panelized building manufacturing is achieved when the maximum amount of work is completed in the factory, with the least waste, in the shortest time. In addition to the studs, headers, and plywood or OSB sheathing, it is possible to install doors, windows, siding, trim, and other finish elements in the factory. However, this creates a heavier and fragile panel which could increase costs and the complexity of transportation.
The process differs from the traditional site-built method because the wall panels are fabricated under controlled environmental conditions, providing higher precision and quality. The site-built method is more labor intensive and more susceptible to errors compared to panelized buildings.
Panelized assemblies are considered to be a green method of construction. The process is recognized by many green building certifications, including the ICC 700 National Green Building Standard. Also, due to its tremendous design flexibility, panelized construction is considered the fastest growing segment of residential buildings in the US.
3.1.2 Process of Manufacturing
Panelized wall components are the same as those used in site-built construction, including studs, top and bottom plates, cripple studs under and above openings, window sill plates, and headers. In both panelized and site-built construction, dimensional lumber, typically 2”x 4” and 3” x 6”, is used for framing. Some manufacturers use finger-jointed studs since they have fewer defects and are more dimensionally stable than solid lumber. The wall sheathing can be constructed using Oriented Strand Board (OSB), particleboard, plaster, gypsum, plywood, hardboard, fiberboard, to name but a few.
As soon as the building design is complete and meets all building codes and special requirements, the wall panels can be manufactured in accordance with detailed shop drawings. The shop drawings include all the basic information for each wall, including the framing and sheathing layout, dimensions, and references to the building plan.
Panel manufacturing is organized in a linear production process, which includes the following steps:
- Lumber is precut using automated computerized machines.
- Sheathing panels are precut.
- Components are arranged on a framing table where framers nail the elements together using pneumatic nail guns. (Fig. 3.2)
- Sheathing panels are nailed to the frame. (Fig. 3.3)
- A router is used to create square openings. (Fig. 3.4)
- Doors and windows are installed, if needed.
- Finished panels are hoisted from the production line, packaged, and bundled together. (Fig. 3.5)
Figure 3.2 Wall panels are framed using precut components.
Figure 3.3 Application of sheathing panels to framing.
Figure 3.4 A router is used to create panel openings.
Figure 3.5 Finished panel hoisted from the production line and stacked with other panels for delivery to the jobsite.
3.1.3 Advantages of panelized buildings
- One of the easiest prefabricated systems for approvals, building codes, lender financing, real estate appraisals and marketing, and neighborhood acceptance.
- The climate-controlled manufacturing environment avoids warping, mold, and other damage caused by weather conditions.
- The precision of automation reduces the errors and miscalculations that are common in the traditional methods.
- All plans are normally engineered before cutting, which eliminates material waste. Small waste elements can also be recycled.
- Reduced time, cost, and labor work compared to traditional methods.
- There is a short learning curve for the architect in learning to design for panelized construction.
- The precision of automated production produces elements with precise dimensions, increased quality, consistent fit, and consequently, good energy performance.
3.1.4 Disadvantages of panelized buildings
- There is a level of inefficiency in transporting pre-assembled panels. Also, if panels must be moved greater distances, they may require additional reinforcement and structure.
- Trucking and transport limitations usually make it much more expensive to work with panels over 8.5 feet in width. Length limitations also must be considered.
- Some panelizers cannot make panels larger than 9 to 10 feet in width.
- The manufacturer must consider standard container dimensions if wall assemblies are going to be shipped.
3.1.5 Case Study: Panel Tek of North Florida, Inc.
Panel Tek produces a variety of structural elements, such as roof trusses, floor trusses, and wall panels. The company provides costumer support during the design phase, manufacturing, material supply, and delivery of assemblies.
Figure 3.6 Left: Covered inventory. Right: Panelized wood walls before installation.
The wall panel production line uses software from MiTek Industries and computerized saws to manufacture wall assemblies. A standard MiTek panelized production line is comprised of assembly and supporting equipment, specialized cutting machines for precise trimming and framing, and pre-cut frame packages (Fig. 3.7).
Figure 3.7 Schematic example of the MiTek panelized production line. This automated production line uses software called eFrame, which designs all the wood elements. The process starts with the component processing center, where the proper material must be loaded in the machine. After that, dimensional lumber is fed to the power framer (2) where panels move through four high production nail guns. A twin-axis sheathing saw (3) cuts the sheathing in X and Y axes simultaneously. A power squaring and sheathing station (4) attaches the sheathing to the top and bottom plates. Finally, a smart crane stacker (5) automatically stacks full levels of 16’ walls and provides built-in fork pockets for easy transport of completed panels.
Chapter 3.2: Structural Insulated Panels (SIP) – Advanced Fabricated Systems
3.2.1 Definition
A Structural Insulated Panel (SIP) is a high performance building system used mostly for residential and light commercial construction. The panels are manufactured under factory controlled conditions in modular sections according to the design specifications. Some manufacturers also provide a ready-to-use paneling system, with openings cut, and the doors and windows installed in the factory. The panels are then transported to the project site, where cranes lift and install each SIP into the correct location.
This system uses an insulated foam core sandwiched by structural panels. Generally, SIP is made of a sandwich consisting of one layer of encapsulated polystyrene (EPS) board between two layers of plywood or Oriented Strand Board (OSB) (Fig. 3.9). However, alternative materials can also be used for both the core and structural skin (Table 1). Cement-board and fiberglass may be used for the structural skin. Normally, gypsum board or other fire-rated material are applied on the inside to minimize the spread of fire. Polyurethane and polyisocyanurate rigid foam can also be used for the insulating material, however they tend to be more expensive due to their better insulation performance, moisture protection, and fire resistance.
Figure 3.9 Left: Exploded Axonometric of a SIPs Building. Right: Traditional SIP panel fabricated from OSB and expanded polystyrene (EPS) insulation.
TABLE 1 TABLE SHOWING THE MOST COMMON MATERIALS USED IN SIPS
Sheathing Material | Major Benefits | Major Disadvantages |
Oriented Strand Board (OSB) | Availability of large and strong panels | Not fire resistant and easily affected by humidity |
Metal | Resistant to mold and can be load bearing | Must be galvanized or stainless steel |
Plywood | Lateral strength | Not fire resistant, easily affected by humidity, reduced structural strength |
Fiber cement siding | Resistant to fire and humidity | High weight compared to other materials and limited panel size |
Magnesium board | Resistant to humidity, fire, and termites | Limited panel size and availability |
Fiberglass or gypsum board | Resistant to fire and termites | Not structural and limited panel size |
Composite Structural Siding panel | Resistant to humidity and termites | Not fire resistant |
Expanded Polystyrene (EPS) | Cheaper; easy to cut on-site; thickness availability | Made with HBCD (hexa-brimo- cyclo-dodecane), a persistant, bio-accumulative, and toxic material. |
Extruded Polystyrene (XPS) | stronger and water resistant | Made of HBCD |
Polyurethane Foam (PUR) | Highest R-value, stronger, and water resistant | More expensive, harder to cut on-site, thickness and availability limitations |
Special attention is required for SIP installation. The main advantage of SIP panels is the airtight envelope they create, therefore, the panels must be carefully placed together. Dimensional lumber is normally used for the connection (Fig.3.10), however it produces thermal bridging and decreases the insulation value. A common practice is to use an insulating material, such as insulated lumber or composite splines (Fig. 3.11). Expanded foam, sealing tape, sealing mastic, and nails are also used for SIP connections.
Figure 3.10 Wall to wall connection for corners or intersections of SIPs using dimensional lumber.
Figure 3.11 Examples of wall-to-wall vertical panel connection.
3.2.2 Process of Manufacturing
The SIP manufacturing process depends on the materials that comprise the panel. For panels made of EPS and OSB, the standard practice is to glue the foam to both panels and compress the resulting assembly to ensure good bonding.
Recently, CAD (computer aided design) and CAM (computer aided manufacturing) technologies have improved SIP production. In this process, CAD/CAM software converts the blueprints of the panel into detailed instructions for a CNC (computerized numerical control) machine, which accurately cuts and produces SIP components.
During the manufacturing process, narrow channels or chases are cut also into the foam for electrical wiring (Fig. 3.12). Electrical wires are then pulled through these channels during SIP installation.
Figure 3.12 Left: Narrow chases or wire chases are cast into the foam for electrical wiring.
Generally, SIP panels are available in thickness from 4 to 6 in. for wall panels, and up to 14 in. for roof panels. Panel size can vary from small to jumbo panels. Jumbo SIPs commonly use OSB sheets up to 8 ft. x 24 ft. Although there are industry standards, SIP panels are customizable in shape, density, dimension, thickness, and appearance for each project.
Figure 3.13 Plan view of a standard production line provided by SIPSTECH, a company which develops technologies for manufacturing processes: In this process the gantry bead glue applicator has the capacity to cover an 8 ft. wide panel of any length. The machine automatically applies glue and water mist. A stack of panels is assembled and pressed in a vacuum press. One cycle can produce 7 panels of 8 ft.x 24 ft.x 4.5 in.. Thicknesses up to 35 in. can be produced.
Figure 3.14 Floor plan of an automated production line provided by SIPSTECH: The system is a single-process machine where materials are placed on one side of the production line and the SIP walls exit from the other side. The machine produces one 8 ft. x 24 ft. panel per 6 minutes, requiring only 2 trained staff. The machine is computer controlled and automatically applies glue to the materials. Glue is not applied to areas where openings will be placed, facilitating the reuse of materials.
3.2.3 Advantages of SIP panels
- The system substantially decreases the time of production, labor hours, and cost.
- Advanced software and automated fabrication technologies allow the maximum material efficiency, drastically eliminating the amount of material waste.
- High levels of insulation provide an energy efficient system, minimizing heat transfer between the inner and outer faces of the building.
- By providing an airtight building enclosure and well-insulated panels, the SIP system allows builders to meet Energy Star requirements, which requires new homes to be 15% more energy efficient than those built to the 2009 International Energy Conservation Code (IECC).
- Most SIP materials can be recycled and reused, a practice that can strongly decreases production costs and minimizes environmental impacts.
- Avoidance of air spaces in the wall that might accumulate moisture and cause mold and other water-related problems.
- SIP panels have better performance compared to framed buildings in extreme conditions, such as earthquakes and storms/tornados.
3.2.4 Disadvantages of SIP panels
- SIPs have the same transportation limitations as panelized elements.
- Cranes are often used for installation, requiring careful project site planning for material delivery.
- Special tools might be needed for on-site modifications.
- A special concern is OSB’s sensitivity to moisture, which must be carefully managed on- site to reduce SIP damage.
- Panel damage or on-site changes can require the entire panel to be replaced, which increases the cost and duration of construction.
- SIPs must not be placed in direct contact with the ground and should be organized in an efficient process that allows the worker to easily read identification marks or labels for each panel.
- SIPs require exterior finishes that protect the structure from water. Asphalt shingles are recommended for a SIP roof. Steel, tile, vinyl, wood, or brick are suitable for siding.
- The insulated foam provides an excellent nesting material for pests. Periodic inspections must be carried out.
- Because SIP panels are practically hermetically sealed and do not allow air exchange, a good ventilation system must be provided for the building to ensure the health and safety of the occupants. Passive air infiltration will not be adequate for good indoor air quality.
- It is recommended that indoor humidity should be controlled by the ventilation system.
3.2.5 Case study: INNOVA Eco Building
INNOVA Eco Building is a company located in Miami, Florida which received the 2016 SIPA Building Excellence Award. The factory uses state-of-art equipment and a conditioned facility to produce SIPs. The company manufactures panels fabricated with different materials, for example:
- Plywood SIP panels: Up to 4 ft. wide and 24 feet length, used for floors, walls, and roofs. The SIP can be made of ½ in. CDX plywood, ¾ in. pressure treated plywood, or other plywood material.
- High Heat SIP: High density rigid polyisocyanurate insulation is used along with magnesium oxide board and phenolic backings to create a high temperature SIP panel for special use conditions.
- Cork SIP: Cork cores are made upon request. Cork harvesting is done by hand without cutting down or damaging the tree. It is 100% natural and rapidly renewable.
- Phenolic Fiberglass: Phenolic skins are highly fire resistant, water proof, dimensionally stable, and accept a wide range of finish coatings.
- Cement fiber skin: Manufactured using an expanded polystyrene (EPS) insulating foam core of 1 to 3 lbs. /cu ft. density, sandwiched between two 5/16 in. thick cement fiber skins. The exterior surface may be painted or coated, if needed.
- Magnesium Oxide Skin: Available in thicknesses from 6 mm to 30 mm. The product is a healthy choice since it does not contain organic solvents, heavy metals, asbestos, oils or other toxic ingredients. Generally, the panel is strong, durable, and impact resistant for hurricane prone areas, not to mention that provides superior loading, fire ratings, and acoustic values over OSB and fiber cement SIPs.
- OSB: Expanded polystyrene insulating foam core of 1 – 3 lbs. /cu ft. density sandwiched between two structural OSB skins. Panels are available in sizes up to 4 ft. wide, up to 24 ft. length, and up to 12 in. thick.
Figure 3.15 Innova production line. Top left: Cement Fiber SIP equipment. Top right: Cement fiber SIP labeled. Lower left: A gantry bead glue machine applying glue over EPS. Lower right: SIP walls assembled, labeled and ready to be transported to the jobsite.
Chapter 3.3 Modular Prefabricated Steel Buildings – Advanced Fabricated Buildings
3.3.1 Definition
Building with steel started in North America in the 1930s. Due to its high cost and lack of flexibility, it has not been a popular method of construction in the residential sector. However, in other types of buildings, mostly commercial, such as manufacturing plants, warehouses, truck terminals, retail stores, and office complexes, it has been a successful construction method. Some reasons for using this method is its durability and the fluctuating level of wood quality. Modular steel building and light-gauge steel stud construction are popular and are widely used in the commercial building industry.
Modular steel can be used in tall, high-performing, or seismic designed buildings. For example, in Japan, they have become widely accepted because the structures are slim and less bulky in comparison to wood. It is strong and rigid, is made with high precision, and is easier to transport. The modules can be produced to near completion with infill, wiring, and ducting, and are able to be rapidly assembled and installed on site.
3.3.2 Manufacturing Process
Fabrication is the process used to manufacturing steelwork components that will, when assembled and joined, form a complete frame. The frame typically uses readily available standard steel sections that are purchased from a steelmaker, together with protective coatings and bolts.
Although a wide range of section shapes and sizes are produced, the designer may find that the required section size is not available. In this case, built-up girders may be fabricated from plates. Sections and plate girders may also be strengthened by stiffening the web or flanges depending upon the load to be carried.
Most modern steelwork fabrication factories have computer aided design and detailing (CAD) machines linked directly to factory floor Computer Numerical Control (CNC) machinery. This means that a computer turns a 3D design into numbers within an X, Y, and Z coordinate system. The three coordinates control the movement and direction of the cutting machine. Using this approach, a volumetric material can be cut in 3 dimensions. The design is produced by Computer Aided Design (CAD) software program. The software is used to produce 2D and 3D precision drawings and technical illustrations. The accuracy of the computer generated details being transmitted directly to the Computer Aided Manufacturing (CAM) machinery increases the quality standards of production. Building Information Modeling (BIM) enables the fabricators to perform scheduling, and cost estimation throughout the process of manufacturing the steel systems.
To increase the speed and efficiency of manufacturing, three types of prefabrication are common: kits-of-parts approach, panelization, and modularization. The advantage of offsite fabrication allows for fast, accurate, and repeatable production. Design and logistical planning play a major role in deciding on the appropriate type of steel prefabrication.
1. A Kit-of-parts Approach:
In this approach, simplification is the key. Here, the components are standardized so they can be easily interchanged to create speed on both ends of the production line and during on-site installation. The advantage of this system is that the parts are demountable, they are able to be disassembled and reused in a different project.
2. Steel Panelization:
Manufacturing light-gauge-steel framed panels offsite allows time and money savings compared to building the individual pieces onsite. This construction system is used for different components of a building such as partition walls and bay flooring, including decking. The panels are built in the factory, shipped, and quickly erected on the site. They can be completed prior to erection, with finished surfaces already preinstalled in the factory.
Figure 3.16 The panelization production system is comprised of 4 steps:
Task plan in the steel panelization production plant
Step (1) Engineering: Drawings are converted by CAD to a list of components that comprise the panels
Step (2) Roll-forming: The data is connected to the roll-formers. The roll- formers create all the pieces with the specification given to them. The material are lifted to panel carts.
Step (3) Assembly: The pieces come together and are attached through a welding and punching system.
Step (4) Finishing: Post welding and sheathing on panels take place. The completed panel is inspected and stacked.
Figure 3.17 The steel prefabricated panel ready to be installed.
3. Steel Modularization:
Subdividing the building volume into smaller units or blocks can save a lot of time by reducing the onsite lifting and installment time. The whole process of building the unit or module takes place in the factory. Afterwards, the module is shipped and is placed and installed. In this construction approach, only minor work is needed to seal the gaps together, and attach the mating surfaces to one another.
Figure 3.18 Technically advanced modular steel installation: The modules can be stacked vertically and horizontally on one another. The floor area can be therefore reduced or increased based on the required program.
Figure 3.19 This alternative designed house, designed by MW Bender Architecture, located in Gainesville, Florida is made of modules, in this case steel shipping containers.
Modular Steel Building Manufacturing Techniques
There are several techniques used in the factory to create a seamless shell, a seismic structure, a weather and water resistant system, and an easy to install system. These techniques are punching, clipping, usage of splices, weather-tightening, usage of flexible ridges, etc.
Figure 3.20 Punching of steel element is done through programming and input by the technician in charge.
Figure 3.21 Punching the steel members in the factory creates a perfect alignment facilitating correct alignment. The holes are not only used to attach the primary framing together but to create a seamless and weather-proof shell. Especially in roof planning, proper alignment of roof panels is crucial to short and long term roof performance. Poor alignment affects weather- tightness and creates problems when installing closures, roof accessories, and trim. (Source: Butler Manufacturing)
3.3.3 Advantages of Modular Prefabricated Steel Buildings
- Steel manufacturing is a highly efficient offsite building system.
- Steel has a high strength-to-weight ratio and is light and strong.
- Steel framing can be used on difficult building sites.
- Steel framing is perfect for long spans, long cantilevers, and large openings.
- It is a perfect solution for flood plain zones because it can be elevated on stilts above water level.
- It is impervious to termites.
- It is a low-maintenance material, especially compared to wood.
- It is flexible for creating curved shapes and forms.
3.3.4 Disadvantages of Modular Prefabricated Steel Buildings
- Steel is expensive.
- Steel lacks flexibility and adaptability for small projects.
3.3.5 Case Study: Butler Building Steel Construction
One of the major manufacturers of steel systems is the Butler Steel Construction Company. They create different systems and types of steel components such as structural framing, roofing, and wall systems. Within these categories, they have various designs that can be customized for many types of projects. Example of these are shown in Figures 3.22 through 3.27.
Figure 3.22 The Widespan structural system allows for maximizing the functionality and productivity of the building’s interior space. The shape of columns can be specified based on the project. The structure is expandable and can be added to over time.
Figure 3.23 The Landmark 2000 structural systems combines solid-web primary frames, factory- punched Truss PurlinXT™ secondary structural members, and rod bracing to form a long-bay framing system that can span up to 60 feet. The columns can be placed up to 60 feet from each other.
Figure 3.24 Multi-story structural systems utilize systems construction to create a cost- effective, high-performance, multistory building. In multistory system design, steel can be used solely as a structural system or combined with other metal wall and roofing components.
Figure 3.25 A non-self-supporting steel frame needs interaction with other construction elements such as precast concrete or masonry infill. A self-supporting steel frame on the other hand is stable and resists lateral loads. Here you see a combination of load bearing walls with steel interior walls and steel roofing system.
Figure 3.26 Storage buildings can be of any width or length, based on 5-foot increments and single or multistory. The benefits of steel framing in storage design is the maximum number of door openings that the structure offers. Also, clear-span interior, fast erection, durability, and low maintenance are some of the design criteria of such a system.
A B
Figure 3.27 A and 3.27 B A metal construction system is used to create small spaces.
Figure 3.28 A conventional metal structure is ready to be completed with metal systems encompassing all conventional steel products — ranging from wide-flange beams and bar joists to pipes and tubes. This production is a good example of the kit-of-parts approach where all parts are standardized.
Figure 3.29 There are different types of roofing systems produced in the manufactured steel plants. The roofing design varies depending on the thermal, daylighting, and remodeling requirements. This is a detail of the MR-24 roofing and a clipping system that allows the metal panels to move responding to the temperature changes.
Figure 3.30 Wall systems can also be customized according to the need and usage of the project. These are examples of Therma-walls used in commercial or industrial building, from architectural applications to cold-storage facilities.
Other case studies
Heavy Structures company is a division of Butler Buildings specializing in prefabricated steel components for very large and complex building projects.
For very large projects where no interruption in space is allowed space-framing can be used. A space-frame or space structure is a light-weight truss system with a uniform geometric matrix that is relatively strong and transmits and spreads the structural tensile and compressive loads. (Fig 3.31).
Figure 3.31 Jacksonville Naval Air Station P302V Maintenance Hangar. To meet the US Navy’s demand for multiple openings for various aircraft, clear spans of 455 feet and 428 feet were necessary at the front of the hangar. To achieve these record-setting clear spans, heavy structures utilized three-story tall box trusses to design the hangar. During erection, these robust trusses were assembled in sections on the ground then lifted into place with the entire system bearing on temporary shoring towers. The structure also supports multiple overhead cranes for maintenance of the aircraft. The 3-D structural design by Heavy Structures proved to be over 25% more efficient than the nearest conventional solution. The size, complexity and economy of this successful project demonstrates the capabilities and advantages Heavy Structures has to offer.
Chapter 3.4 Precast Concrete Buildings – Advanced Fabricated Buildings
3.4.1 Introduction
In general, concrete is used in many forms and for various types of construction: concrete block, concrete panel, cast-in-place concrete, and recently, hybrid forms of concrete mixed with fiberglass, carbon, or steel fibers. Precast concrete construction is the casting and production of concrete components offsite and shipping them to the site for assembly into a building.
There are two major categories of precast concrete based on its physical appearance and usage:
- Architectural precast concrete remains visible and exposed after erection contributing to the architectural finished effects such as shape, color and
- Structural precast is covered by other elements and is mainly used for structural purposes.
Precast compared to onsite poured concrete has better quality, higher production control and monitoring. It is environmentally friendly because the formwork is meant for multiple use and saves valuable production time.
Figure 3.32 Precast slabs and beam shapes
Shapes
The common precast shapes that are available in the market are listed in the table below:
TABLE 2 COMMONLY PRODUCED PRECAST CONCRETE SHAPES AND SIZES
Precast Element | Specifications | ||
Width | Depth/Span | Supporting Area | |
Solid Flat Slab | varies | 1/40 of span ranging 3.5 to 8 in. | N/A |
Hollow Core Slab | 2, 4, and 8 ft. | 8 in. /25 ft., 10 in. /32 ft., 12 in. /40 ft. | N/A |
Double Tee | 8 ft., 10 ft. | 1/28 of span, depth include 12, 14, 16, 18, 20, 24, and 32 in. | N/A |
Single Tee | 8 ft., 10 ft. | 36 in. /85 ft., 48 in. /105 ft. | N/A |
Beam & Girders | light loads: 1/2 of depth | 1/15 of span | N/A |
heavy loads: 1/2 of depth | 1/12 of span | N/A | |
Columns (square) | 10 in. | 10 in. | 2,000 SF |
12 in. | 12 in. | 2,600 SF | |
16 in. | 16 in. | 4,000 SF | |
24 in. | 24 in. | 8,000 SF | |
Spandrels, Cladding, Walls | varies | varies | N/A |
3.4.2 Process of Manufacturing
The process of producing the pieces starts by designing the components in a CAD program and transferring the 3D model to the plotting stations in the manufacturing plant. Formwork is mostly fabricated from steel and is formed into pans or separators assembled as a negative shape of the final product. The formwork is robust because it is highly loaded then disassembled and interchanged for reuse in the factory.
Figure 3.33 Steps to produce precast concrete components in the manufacturing plant
At this stage, the cast-in items such as pipes, electrical boxes and conduit are set in the formwork which is sealed to keep the liquid mixture within. Also, other connecting devices such as anchorage, reinforcing steel bars and braces are laid inside the form to perform structural stability and to create connections to be used at later stages of installment. The reason that steel reinforcement bars are used together with concrete is to create strength and to prevent the positive and negative bending and cracking. (Fig. 3.33)
Meanwhile, the raw material consisted of aggregate (sand, rock, or gravel), water, and Portland cement are mixed and ready for pouring into the formwork. After applying a coat of oil to the inner surfaces of the formwork, the batch is poured into the forms and vibrated to release the air bubbles trapped in the mixture. Next, the surface is leveled and screeded to create a finished surface. To screed is to level a floor or layer of concrete with a straight edge using a back and forth motion while moving across the surface. The concrete is now ready to be cured where heat is applied in specific areas or chambers. After the curing time is complete, the piece is ready to be demolded and detached from the formwork.
The structural design requires the performance and behavior of the final elements. There is usually a testing procedure in the factory right after the offsite production is complete. However, the result of testing in that stage is not sufficient because there are other stresses that will be applied before final installation. These stresses include the handling of the material such as lifting, transportation and erection. A post-testing is additionally required for structural purposes which should be considered for the final stages in the process.
Attachment Methods for Precast Concrete Components
Connecting the precast concrete units together and to the main structure is a major design aspect of precast concrete assembly. The stability of the building as a whole and its safety, performance and economy of use in general are the outcome of such an assembly. Components such as wall panels, facings, interior partitions, columns, floors, hangers, and other precast elements are attached to each other and to other non-precast members by a combination of different connecting pieces such as fasteners, seats, brackets or outriggers, shear plates, tiebacks, bolts, welds, anchors, and inserts using different kinds of methods. The main 3 attachment methods are explained below.
1. Embeds
An anchor bolt or anchor with weld plate is cast in the factory within the precast element. Templates are used to place embeds while the concrete is wet. After the concrete is ready, the embedded surfaces will be welded together with other steel elements such as loose plates or angles. (Fig. 3.35)
Figure 3.35 Bolts of various shapes (L, J, and pig-tail) are embedded or anchored into the liquid concrete.
2. Epoxy set (Post-installment)
This method requires drilling and cleaning a hole and then placing a bolt or hanger that is then set with anchoring cement, lead grout, or a thermoset polymer such as epoxy. (Fig. 3.36)
Figure 3.36 After the concrete has set, bolts and epoxy glue are applied.
Considerations: (1) The hole where the screw go should be cleaned. (2) The epoxy glue to be mixed in the exact amount. (3) The set time has to be precise. (4) A testing procedure has to be carried out.
3. Expansion anchor (Post-installment)
This method also requires predrilling. An expansion anchor is placed into the cavity. The bolt, screw, or hanger is then placed within the anchor and, when engaged, expands to restrict its movement. (Fig. 3.37)
Figure 3.37 After screwing and tightening, the expansion anchor widens in diameter and creates a tight connection. (Source: Cobra Anchors)
3.4.3 Advantages of Precast Concrete Buildings
- Precast concrete is usually less costly than onsite concrete
- Availability of raw materials
- Flexibility to create complex shapes
- Natural sound barrier
- Ability to be cut and drilled
- Weather conditions do not affect the offsite
- Curing time on site is not
- In architectural precast application, no additional material
- No shoring required
- Immediate backfill after installation
- Unlike onsite, formwork is not site
3.4.4 Disadvantages of Precast Concrete Buildings
- Limitations on producing large sizes (up to 14 feet in width) due to truck carriage size and regulations
- Additional features such as bolts, reinforcing bars, and mesh have to be
- More testing is required
- Reinforcement considerations whether it is conventional or pre-stressed steel
- Heavy compared to wood and steel construction
- Structural performance may be affected due to lifting, transporting and
3.4.5 Case Study: FINFROCK Industries producing Precast Concrete
FINFROCK is a producer of precast/pre-stressed concrete building system located in central Florida. This company’s turn-key projects complete all stages of work from design to installation of the sections.
Their range of responsibility includes architectural and structural design, 3D modelling, building information modeling (BIM), construction and permit documents, scheduling, MEP/FP design and sub-consultant management, parking and traffic consulting, feasibility studies, site evaluations, manufacturing, installing, quality assurance, supervision, certificate of occupancy, project close-out and even maintenance and repair.
The components are flexible and can be integrated into other subsystems such as mechanical, plumbing and electrical.
Figure 3.38 Dania Beach Park-N-Ride Parking Garage, Dania Beach, Florida. This garage, completed in 2010 by FINFROCK, serves passengers for the Tri-Rail high-speed rail line that serves Broward, Miami-Dade, and Palm Beach Counties. It has 402 stalls, and two stories.
Figure 3.39 After winning the contract for the Venture residential project, the contracting company approached FINFROCK to assume responsibility for the design and construction for the 720-stall parking garage portion of the project. FINFROCK served as architect of record, structural engineer, design-build contractor, precast concrete manufacturer and erector, and parking consultant for the project.
Chapter 3.5 Manufactured Components: Truss Fabrication – Advanced Fabricated Buildings
3.5.1 Definition
A truss is a triangular system of interconnected structural elements that allows more structural support using less material by distributing the load. Trusses are commonly used in buildings and bridges. In buildings, trusses are used to support floors and roofs.
Figure 3.40 Diagram of a basic truss indicating the names of the different members.
Dead refers to the load that remains same and does not change over time, such as the load of materials and the components of the structure itself, like weight of framing, roofing material etc. In manufactured homes, trusses are used to take the dead load of the roof structure and other loads such as snow load and wind load. There are different types of trusses that can be constructed or manufactured with different materials such as steel, wood, concrete and other metals. The type and materials of the truss are determined based on the span, type of structure, and on the load on structure. Trusses are installed continuously along the exterior wall at pre-determined intervals to support the total load of the roof.
Figure 3.41 Examples of commonly used trusses. Trusses are usually installed one after the other, at a fixed interval designed based on structural requirements.
Most manufactured home companies use prefabricated roof trusses. Prefabricated roof trusses are trusses built in a factory and carefully designed to carry the load of roof to the exterior walls. They are shipped to the manufactured home factories and installed in the homes at a predetermined interval using a crane.
3.5.2 Advantages of using truss system
- A roof truss system allows a light weight roof system and trusses can be designed for any shape imaginable.
- Ceiling styles such as cathedral ceilings are less expensive using
- A truss system allows a much longer span without the need for load bearing interior walls.
- Manufactured trusses are designed by engineers to meet building codes, enabling more uniform and accurate
- Manufacturing inside a controlled factory setting ensures that the materials are not exposed to weather and moisture conditions. Thus a better quality product can be achieved.
- Delivered in bulk on site, trusses can be installed in a single day, speeding the process of construction.
- Trusses are cheaper due to bulk manufacturing, and less
3.5.3 Disadvantages of using truss system
- Transportation of trusses can be a problem as trusses can be bigger than the truck in some cases.
- The webbed structure of trusses leaves a large volume of attic space
- Wooden trusses are susceptible to